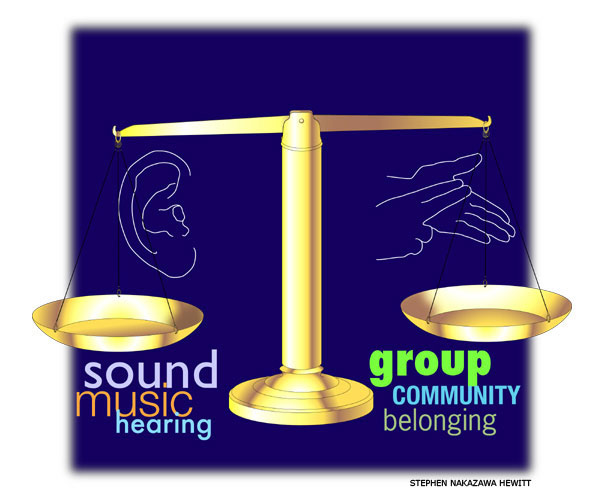
A single ear pokes out from a mound of crisp blue hospital dressings. It belongs to a patient lying on a table in the middle of a bustling operating room. Drill bits of all sizes lie in neat rows next to scalpels and clamps on a sterile table. Surgeon Lawrence Lustig bounds among a whirlwind of nurses in blue and beige as he gets ready to bore into the patient’s skull. “We’re going to stay out of the brain today, we hope,” he says, grinning.
The patient, a man in his fifties, is deaf. But one month from today sound will penetrate his silence when he activates the electronic implant Lustig is about to thread into his inner ear. As director of the Douglas Grant Cochlear Implant Center at the University of California, San Francisco, Lustig has performed hundreds of these surgeries, managing to stay out of the brain every time. He still marvels at the result: The deaf are able to hear.
Cochlear implants are more than hearing aids. They actually bypass the ear to transmit sound signals directly to the brain. Since their introduction in the 1970s, they have brought sound to thousands of deaf children and adults, and steady improvements in technology have made the latest implants dramatically more powerful than the early models. Today, many recipients can have normal conversations and talk on a cell phone. Others, however, don’t do as well, and there is debate about why. Current devices also cannot handle the intricacies of music. But even that problem may soon be solved as advances in design promise to refine the implant’s communication with the brain, allowing the deaf to appreciate melody.
Lustig’s enthusiasm for this technology is contagious. “To me it is so completely fascinating that we can take a sense that was lost and replace it, even only partially,” he says. “It’s the first time we’ve ever been able to do that.”
A Cure for Deafness?
In 1957 a French doctor, at the insistent request of a deaf patient, inserted the first crude implant into the inner ear. It was a simple wire electrode used in physiological research. The idea was to electrically stimulate the auditory nerve, giving the deaf person some perception of sound. Though the patient was disappointed and the implant was removed, the theory had promise. By the 1970s, the burgeoning fields of materials science and microelectronics gave cochlear implant research the boost it needed to move from the theoretical to the experimental.
Hearing loss usually results from damage to the cochlea, the spiral-shaped, fluid-filled cavity in the inner ear. A forest of tiny hair cells inside the cochlea passes sound to the auditory nerve by changing vibrations into electrical impulses. The cells at the base of the cochlea vibrate at high frequencies, while ones at the top respond to low frequencies. The brain interprets the frequencies based on which cells within the cochlea trigger the nerve.
The early researchers needed to create a device to do the cochlea’s job. They invented a tiny curved wire, dotted with electrical contacts and coated in clear silicon. It looked like a mysterious deep-sea creature. This electrode array slid deeply into the cochlea to send electrical impulses directly to the auditory nerve, bypassing the damaged hair cells. An external ear piece picked up sounds and turned them into electrical signals to send to the array. Current implant systems are essentially the same.
But early inventors had a problem. Humans have about 3500 hair cells in each ear. And each cell has up to 30 nerve endings that feed the auditory nerve. Scientists did not fully understand how those cells sorted out sound, especially speech. This ignorance spurred strong opposition to the first experimental trials.
“The prevailing attitude at the time, of all the premier auditory scientists in the field, was ‘Based on everything we know, this can’t work,’” says Lustig. “And they were absolutely right. But a couple people just kept plugging away at it, and it ended up actually working.”
The first devices had one channel, meaning they could only stimulate the auditory nerve at one place along the cochlea. This severely limited the frequencies a patient could hear. By the mid 1970s, however, multichannel devices were developed that activated the nerve at several places. These delivered a range of frequencies and allowed people to comprehend some speech.
“The multichannel devices rocketed the whole science forward,” says Lustig. By the mid 1980s, the U.S. Food and Drug Administration (FDA) had approved two devices for use in adults. The FDA slowly reduced its restrictions over the next 20 years, allowing many more people to qualify for implantation. Now, children as young as 12 months can receive implants.
This boom in the field of cochlear implants met opposition from a surprising source, however: the deaf community. With an entire culture centered around not being able to hear, they saw this new technology as a threat to their way of life. (See To be deaf or Deaf)
Today that friction is lessening, and implants are becoming more common. The best current implants boast up to 22 channels and cutting-edge voice recognition software. Despite the advances in technology, however, success varies tremendously among implant recipients. Extensive rehabilitation, where a patient must learn how to listen, is often necessary. And of course, the surgery has risks.
Patient by patient
The operating room smells like a barbecue gone wrong. After slicing a four-inch opening behind the patient’s ear, Lustig and a resident, Dr. Doris Lin, scrape away the lower layers of skin, cauterizing as they go. Amid a smell of burning flesh, they test to see whether the space they created is big enough for a flat, rubbery transmitter. This device sits under the skin, receiving radio signals from the external ear piece and relaying them to the electrode array inside the ear. Satisfied with the fit, the doctors begin drilling straight into the skull behind the ear. The bone dust flying, they work their way down to the middle ear. At a certain depth, they stop. They swing a large microscope into position over the patient’s head. The next part is the riskiest and they need to see exactly where the drill is going.
Lustig and Lin now stare intently through the eyepieces. They must find the opening to the cochlea without damaging the nerves and bones in the middle ear. As Lustig gently guides his resident through the final drilling, he notices the facial nerve has been exposed. “We can’t hit that or we’ll give him permanent facial paralysis,” he explains, pointing out the nerve on the microscope monitor as Lin continues to drill. Another doctor watches the patient’s neural activity on a monitor and reports any hiccups, warning the surgeons if they get too close.
As risky as this seems to an outside observer in the operating room, the chance of paralysis is small and fails to deter hundreds of people who seek implants every year in the U.S. Lustig alone performs or oversees about one surgery every week. But the degree of success is a difficult thing to measure, says Colleen Polite, an audiologist at the Douglas Grant Implant Center at UCSF.
“Success is really based on your point of reference,” she explains. To some, success means being able to hear environmental sounds, or being able to read lips more easily. “For other people success means they can talk on a cell phone,” she says. But either way, she observes, the implant “changes people’s lives dramatically.”
Polite describes one patient who came back to her office after using the restroom, utterly shocked to find public restrooms were not private. “She said ‘I could hear people washing their hands at the sink, I could hear them flushing the toilet! I didn’t know other people could hear me in the bathroom!’” Other patients report similar surprises. One woman was amazed she could hear her cat drinking out of its water bowl. “To her, it was the most beautiful sound she’d ever heard,” recalls Polite.
Neil Kran, another of Polite’s patients, is the ultimate cochlear implant success story. Diagnosed with severe hearing loss at age five, his condition worsened progressively. Around age 30 he lost the ability to communicate. He became part of what he calls the “nether world:” the community of late-deafened people who are not part of the deaf culture and do not have the associated skills and support. Like many in this world, he had difficulty dealing with his hearing loss. But then, Kran joined a group called Hearing Impaired Professionals, where he met several cochlear implant recipients. Four years ago, he finally decided to receive one himself.
“How many times can you say ‘what?’ to your wife?” he says. “And you’ve got your colleagues at work and other friends. After a while you really start withdrawing.”
Now, however, he can use a cell phone, carry on a normal conversation in a bar, and hear birds in the trees. But the change wasn’t instantaneous. The implant introduced a whole range of frequencies he had never heard, even as a child with a hearing aid. He had to adapt to these new sounds.
He describes the first time he opened the New York Times after his implant was activated. “It was like the fourth of July, bombs and firecrackers going off! It was a sound, a frequency range, that had no place in my head to be recognized,” he explains. Over time his brain adapted, however, and now he says he doesn’t even notice the sound. “The brain is very plastic,” he says. “It decides for you what’s important and what’s not.”
As Dr. Lustig puts it, “The brain’s an amazing organ. It’s much smarter than we are.”
But patients do need to help the brain. Listening practice is vital, says Kran. He used English as a Second Language CDs to practice listening to speech. Others use books-on-tape or listen to someone reading. Many environmental sounds need explaining too, even for people who used to hear.
This rehabilitation process is closely linked with programming the speech processor. The first thing someone hears when the implant is activated is a beep coming from the audiologist’s computer. Based on the patient’s responses, the processor is tuned to transmit certain frequencies at certain intensities, much like adjusting a graphic equalizer on a stereo system. Then, as the brain adapts, the patient returns every few months for reprogramming.
Even with all this programming and rehabilitation, however, some patients don’t do well. The person’s history accounts for most such cases, say Lustig and Polite. Adult patients who were born deaf and never learned to speak usually have the least success with cochlear implants. Not surprisingly, those who went deaf later in life, after learning to speak, do better. The best patients, however, are children born deaf and implanted early, when the brain is most plastic.
But some in the medical research community say many failures arise from faulty electrode implantation. The Epstein Laboratory in the Department of Otolaryngology at UCSF – where one of the first electrode arrays was created – now focuses on the physiological effects of implantation. A recent study that used cadavers to look at the results of electrode insertion suggests that as many as one-third of the arrays may be inserted wrong, either because of surgeon error or unusual anatomy. The cochlea has three sections, only one of which is suitable. Many of the arrays went into the wrong section. Others broke through the internal membrane and ended up in the wrong section, causing significant trauma.
Russell Snyder, a research scientist in the Epstein Lab, thinks misplaced arrays, rather than the patient’s history or psychology, explains most unsuccessful cases. “They go where they happen to go as the surgeon puts them in, and they rely on the cochlea for guidance. It’s like putting a coat hanger down the drain,” he says.
Onward and Upward
With the opening to the cochlea found, Lustig takes the driver’s seat. “I promised the patient I’d do the actual insertion,” he says. He picks up the $20,000 implant system, slipping the transmitter into position under the skin and grasping the long probe with the electrode array at its end. The room gets quiet as he slowly feeds the probe into the man’s head. As he works, he softly describes to Lin what he’s doing. Finally, he sits back and points to the monitor, where the tiny silicone-coated wire emerges from the inner ear. “There it is. There’s the implanted electrode,” he says.
Lustig claims surgery is the easiest part of the process, but not all surgeons have his experience with the procedure. New advances could greatly improve the delicate insertion. Researchers at the University of Michigan have developed an implant with sensors that tell the surgeon when it brushes the wall of the cochlea. UCSF scientists are also working on an electrode array with sensors, Snyder says. “You’d like to give the surgeon some feedback as to what the electrode is doing, because all he has is a hole,” he notes.
Most of the latest implant research, however, is aimed at improving the perception of music. Due to a limited number of channels, current implants have trouble picking up music’s essential frequency changes. Researchers in the Epstein Lab are studying the possibility of creating virtual channels. By dividing one signal between two electrodes at different points along the nerve, a cross reaction can occur that stimulates nerve endings in between. “By doing that, some studies suggest, you could get up to 100 different channels and therefore start getting a much truer delivery of sound,” says Lustig.
And researchers at the National Physical Laboratory (NPL) in the United Kingdom have built a prototype for a completely new type of implant: one that would pick up the subtleties of music. The device consists of tiny bars tuned to resonate at different frequencies. The bars are coated in a piezoelectric material, which produces a voltage in response to movement. The device would be completely implantable, with no external parts or batteries.
“Sound waves would come into the ear and generate the voltage directly inside the cochlea,” says Bill Nimmo, business manager for the Functional Materials Group at NPL. “The vision is to produce something that is almost like a contact lens for the ear.” The current prototype is too big, and the researchers are trying to miniaturize the device. Nimmo says the final product could have 100 bars, each acting as a different channel to greatly enhance music appreciation. It could transform the field of cochlear implants, he says.
Meanwhile, the makers of current implants are trying to broaden their market by creating hybrid devices. These combine natural hearing with electronic hearing for people who have gone partially deaf, usually due to old age. Simply a shorter version of the current model, the implant sits in the base of the cochlea to stimulate the high frequencies, those typically lost in later life. The working hair cells at the top of the cochlea are left alone. The Douglas Grant Center has started to implant these as part of a series of clinical trials, says Lustig. If they work, millions of people could qualify – aging baby boomers being the most obvious candidates.
With more people in line to receive implants, Neil Kran wonders about the technology’s ultimate reach. Bypassing a sensory organ to directly stimulate the brain “opens up all these wonderful possibilities,” he says.
A scientist himself, he wonders about the limits to the brain’s sensory
abilities. Dogs can hear frequencies humans can’t, and other mammals can
smell 10,000 times better than we can, he muses. We’re all related
evolutionarily speaking, so perhaps our sensory organs are holding us back;
perhaps our brains could sense things beyond our imagining. “One day, maybe
they’ll really extend that capability,” says Kran. One day, maybe everyone
will want an electronic ear.
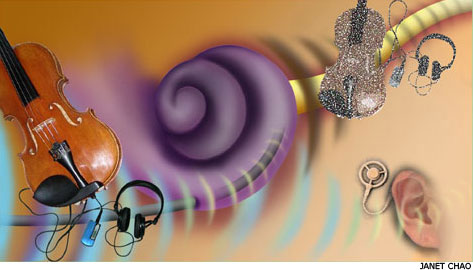
To be deaf or Deaf: the deaf community’s reaction to cochlear implants
Being Deaf with a capital “D” is very different than merely not being able to hear. To be Deaf is to belong to a culture, with its own schools, communities, entertainment, and of course language: sign. When the first cochlear implants began to receive recognition from scientists, deaf leaders spoke out strongly against them. The technology, they feared, would eradicate their culture.
“The deaf community doesn’t feel that deafness is a disease,” says implant surgeon Lawrence Lustig. “They don’t feel they need to be cured.” Instead, many in the community saw attempts to cure deafness as a form of oppression. They also strongly objected to giving implants to children, since the child does not make the choice, and the long term effects of implantation are unknown.
In a 1993 statement, the National Association of the Deaf (NAD) vehemently opposed the Food and Drug Administration’s approval of the device for children as young as two years. The authors did not believe implants helped a child’s psychological and social development, and they wanted parents to know more about the benefits of becoming part of the signing deaf community.
A letter to the editor in the September 20, 1999 edition of the Savannah Morning News, a Georgia newspaper, illustrates the deaf community’s concerns. The 19-year-old writer says he felt “uncomfortable and stressed out” after receiving an implant when he was 10. “In my opinion, a cochlear implant is close to child abuse. Implanting a deaf child is a way of not accepting who your child is.” Though some still feel this way, the deaf community has largely accepted the devices are here to stay. The NAD’s current position statement on cochlear implants acknowledges the importance of diversity in the deaf community, and extends that to include implant users. “It is a tool, just like hearing aids – except this high-tech device requires surgery to implant,” says Bridgetta Bourne-Firl, outreach specialist for the California School for the Deaf in Fremont. “The surgery seems invasive and unnecessary to some. To others, it is something that just needs to be done, as a form of cure or logical medical intervention. Whether someone has a cochlear implant or not, the most important thing is how they see themselves as a person, how well they connect to others. A cochlear implant should and must not define a deaf person. It is a tool.”
ABOUT THE WRITER
Aria Pearson
B.A. (integrative biology), University of California, Berkeley
Internship: Wired
I always wanted to be a marine biologist. After a year and a half spent manhandling sea lion fecal samples and rooting around inside fish heads looking for tiny bones, I still wanted to be a marine biologist, though with less gusto. When I stumbled across the SciCom website it was like being slapped in the face. The exhilaration I felt made me wake up and realize that, though I loved science, my enthusiasm for becoming a scientist was lukewarm at best.
Science is everywhere and cannot be ignored. Communication is the key, capturing people’s attention and inspiring them to think critically about their world. I can think of nothing more fulfilling and worthwhile than spending a lifetime chipping away at the walls of fear and misunderstanding that stand between too many people and the world of science.
ABOUT THE ILLUSTRATORS
Stephen Nakazawa Hewitt
B.S. (physical science), Warner Pacific College, Portland, Oregon
M.S. (microbiology), Oregon State University
Stephen went to graduate school to become a scientist. He learned a lot,
and he loved it. But, during the process, he realized that there is a
dearth of sufficient educational tools for students. He truly feels that
the dissemination of scientific information is just as important as its
collection. In order to clarify class concepts, he spent hours diagramming
the molecular pathways and protein-protein interactions he was studying.
As a result of these figures, others in the lab asked him to do
illustrations for them. From this experience, he realized how important
the formulation of succinct figures is to eloquently elucidate ideas. In
order to gain these tools he applied to the Science Illustration Program.
His hope is to join artistic skills gained from this program with a
background in science, to convey research that scientists want illustrated.
Janet Chao
B.S. (bioengineering; minor in chemistry), University of Illinois at
Urbana-Champaign
Janet Chao was born in Taiwan, and her first experiences with art involved
the traditional crafts of that country. In 2005, she received a B.S. in
Bioengineering from the University of Illinois at Urbana-Champaign. Looking
for a way to combine her interests in art and science, Janet turned to the
Science Illustration Program at U.C. Santa Cruz. Now, certificate in hand,
she is preparing to start her career with an internship at Scientific
American magazine. Janet has illustrated a new species of fish for an
article in Coepia, different varieties of lettuce for The Cultivar, and the
nerves of the human arm for Bell Sports. She is fascinated by the
intricacies of the human body, and would like to eventually focus on
illustrating medical subjects. Her Web site is http://www.illustrationideas.com/.