![]() |
OVER THE COURSE of a year, according to one study, a single barn owl eats approximately 115 meadow voles, 226 pocket gophers, 167 white-footed mice, 83 house mice, 3 kangaroo rats, and 101 roof rats—along with occasional starlings, moths, crickets, and frogs. Not impressed? Consider this: Most of these critters were caught in total darkness.
Barn owls can pinpoint the location of a noise with such precision that even on a moonless night, the rustle of leaves can be a scampering rodent’s death knell. Like most animals, soon after birth, owls are able to recognize where a sound is coming from. As they grow up and go through the motions of hearing and catching their prey, the networks of neurons in their brains that interpret sound becomes increasingly refined, reinforced by the experience of hunting. In the parlance of those who study how the brain learns, this window of time when neural connections can still be calibrated by experience is called the sensitive period. Scientists have long investigated sensitive periods in the context of research questions ranging from how babies pick up a language to the development of binocular vision in young mammals. Until recently, conventional wisdom said that little could be done to extend the brain’s “plasticity” once the sensitive period had passed. The adage about old dogs actually has a basis in neuroscience. Now, however, research is challenging that notion. Stanford University neuroscientist Eric Knudsen is using the barn owl’s exceptional aural ability to explore how and when brains do their best learning. For the past 30 years, he and his colleagues have been untangling how the bird’s brain crunches incoming sensory data to create and synchronize mental maps of both the auditory and visual world. The twists and turns through the owl’s neural circuitry have recently led Knudsen to a surprising discovery. He’s finding that even after the critical window of early learning closes, an old brain that is fed information in the right way can still learn new tricks. The key, it turns out, is in the packaging. When new information is received in small doses, or in an especially stimulating environment, the brain is much more likely to incorporate it. These findings are consistent with recent studies of how infants learn language, and suggest promising strategies for enhancing learning ability in adults. Knudsen has been investigating how the brain processes information since he was a graduate student at the University of California in San Diego in the late ‘70s. His early studies focused on catfish, which have inner ears that hear vibrations in the water and also detect the electric fields of nearby living things. Knudsen found that the catfish brain processes these two types of sensory information—auditory and electric—in separate, but parallel, pathways. That research raised a question: “I wondered, is the auditory system in terrestrial animals organized the same way?” Knudsen recalls. “Does it separate out what a signal is from where it is located in space?” Sipping from a John Deere coffee mug in his Stanford office, Knudsen explains how his research focus moved from catfish to owls. Tall and blond, with piercing blue eyes, he looks more like a Norwegian bachelor farmer than a neuroscientist. He gestures at a poster on the wall, a stunning time-lapse photograph in black and white: A barn owl, its wings and talons extended, appears against a midnight black backdrop, swooping down towards an unseen mouse. A colleague, neuroscientist Mike Konishi, took the picture with an infrared video camera. “It was basically a completely dark room, and he just opened the shutter and strobed an infrared light,” Knudsen explains. “He’s showing how the owl uses hearing to find its prey.” Knudsen was finishing his Ph.D. when he first heard about Konishi’s investigations of barn owl behavior. Konishi, who had video-recorded barn owls reacting to sound in a totally dark room, was struck by the speed and accuracy with which the bird turned its head towards a noise—its response was as swift as the flick of a human eye shifting its gaze. Knudsen realized that the owl’s auditory prowess would make it an ideal study subject for investigating how the brain processes sound and integrates it with visual information. Not long after the two met at a conference, Knudsen began a postdoctoral fellowship working with Konishi at the California Institute of Technology in Pasadena. Their early experiments showed how the barn owl, like humans, merges the information from its two ears to locate a sound. By comparing differences in the timing and intensity of, say, a mouse squeak or rustle of leaves reaching the two ears, the bird’s brain makes a rapid computation that pinpoints the source of noise—and calculates the precise flight trajectory that will land it dinner. The differences in the auditory information that reaches the two ears are amplified by the architecture of the barn owl’s head. The bird’s face is asymmetrical: Its left ear, which sits higher on the face than the right, is surrounded by feathers that are oriented downward, enhancing sounds from below. The inverse is true for the right ear, which is more sensitive to sounds from above. The birds’ filamentous facial feathers are arranged in such a way that they form the equivalent of satellite-receiving dishes on either side of the beak. These facial discs collect sound, focusing it towards the ears. In the late 1970s, Knudsen and Konishi began a series of experiments hoping to pinpoint which neural networks in the owl brain distinguish where a sound is located. The researchers first looked at the forebrain—the seat of higher, complex functions in vertebrate animals—but soon moved on to the midbrain, a region responsible for orienting the eyes and ears to important events in the environment. After connecting tiny electrodes to the anesthetized brain of a barn owl, they moved an audio speaker around the bird’s head while playing a variety of noises, and tracked the neuron firing patterns. To the investigators’ astonishment, the pattern of firing changed dramatically. “The neurons were really sharply tuned for sound location,” recalls Knudsen. “They didn’t much care about how loud the sound was or what kind of sound it was, but they cared very much about where it was coming from—it was pretty surprising.” Scientists had known that the vertebrate brain builds a visual map with information coming from the retina, but how it interprets auditory information was largely unexplored. Like a surveyor, the owl’s brain was taking measurements of sounds and crunching the numbers to map the outside world of sounds depicting actual space, Knudsen says. “The auditory system was going to great lengths to create a representation of space that was very like the visual representation, even though it was starting with very different information. It was mapped topographically—very much like a regular map, and very much like the visual map.” With his own graduate students at Stanford, in the late 1980s Knudsen found that this auditory map is sent to a specific region of the midbrain, the optic tectum, where it is aligned with the visual map. So when an owl hears a mouse in a certain location, a cluster of neurons fires in a specific area of the optic tectum; and when it sees the mouse in the same place, neurons in that area are triggered again. THE DISCOVERY of this combined visual-auditory map led Knudsen to pursue another question, he says: “How much could we distort the sounds, and still have the owl accurately reconstruct the world in its head?” To find out, the researchers took advantage of a quirk in barn owl anatomy. The eyeballs of these creatures actually aren’t balls at all, but elongated, piston-like tubes. Pulling a barn owl skull off a bookshelf, Knudsen explains how each empty eye socket has a cylindrical structure that gives the birds highly sensitive vision. But the eye’s mobility is relatively fixed. To move its eyes to the left or right, the bird must turn its head. In fact, some species can rotate their heads 270 degrees and look behind them. Upon hearing a noise, an owl instinctively looks for its cause. If the sound is coming from anywhere but directly in front, the bird must swivel its head to see the source—and it does so as quickly as the human eye darts to an unexpected noise. Because head movements are much easier to see and count than the flicker of an eyeball, Knudsen could test whether the owl’s audio and visual maps remained in sync when he exposed the animal to distorted sensory cues. He and his colleagues manipulated the auditory information reaching the bird’s brain by stopping up one ear with a plug. Then the researchers compared neuron-firing patterns in the hearing-impaired birds versus the normal birds. Initially, the birds with an earplug misjudged the location of a sound, repeatedly swiveling their heads to look for a noise source off to one side when it actually was straight ahead. But over a period of weeks, something remarkable happened. The owls’ auditory maps actually compensated for the earplugs, so they accurately oriented to the sounds. These behavioral experiments with the earplugs provided the first evidence that a sensitive period might influence the birds’ ability to adjust the brain pathways from noise to neuron. “We had no idea that there would be a developmental component to learning in the auditory pathway,” Knudsen says. “But the young birds were able to make sense of the altered cues caused by the earplugs very easily, and the adult owls could not.” Alfredo Kirkwood, who studies neural plasticity in rats at the Mind/Brain Institute at Johns Hopkins University, says the results make sense when cast in a developmental light. “You want the plasticity when the body is growing. When your head and eyes and ears are growing, you have to adjust all the time to keep the sensory system working with high precision,” says Kirkwood. Much of the brain’s circuitry is established before birth, guided by genetics. Since many of the interactions between body and surroundings are predictable—pain is bad, for instance, whereas food is good—the nervous system has evolved to cope with an environment that is, in many ways, consistent. But many neural connections remain malleable, allowing the brain to adapt to the body’s environment. While that developmental window is open, experience dramatically shapes which neural connections are established in the brain, and the networks laid down during this period are strong. As we age, however, those networks become much more difficult to adjust. For instance, the neural connections between the eye and the brain’s visual cortex normally grow stronger during early development. Landmark experiments showed that kittens with one eye blindfolded at birth never strengthened those networks—and when the patch was removed in adulthood, the eye was permanently blind. “As the brain matures you get specificity and lose plasticity,” says Kirkwood. KNUDSEN’S INVESTIGATIONS of the barn owl noggin next delved into figuring out which mental map the brain trusts when its audio and visual systems aren’t in sync. In a set of experiments in the ‘90s, Knudsen again distorted the auditory signal with an earplug, and once the birds had adjusted to the situation, he put blinders over their eyes—little spectacles covered in masking tape. Curiously, the results showed that once the earplug was removed, the owls that were wearing spectacles couldn’t relearn the original, correct map for sound. “They got stuck with the altered map,” he recalls. Somehow, the visual system was overriding and correcting the auditory system with what Knudsen calls an “instructive signal.” “The only time the signal raises its head is when there is disagreement,” Knudsen says. While very little is known about the instructive signal, the findings suggest that over the course of evolution, sight has proved a more reliable indicator of spatial information than sound. The implications are powerful, he says, because this executive order from the visual system seems to drive changes in neural plasticity, telling the brain when it’s got it right. “If we can figure that out, then we can exploit it,” he says. To find out more, Knudsen tried a different experimental strategy. By manipulating only the birds’ visual information, he found he could shift their response to sound. Knudsen fitted his owls with goggles made of special prismatic lenses that shifted their visual world 20 degrees horizontally to either the right or left. In a dark room he exposed the birds to a sound stimulus, a burst of noise from a loudspeaker positioned off to the side. Initially, trying to look directly at the sound, the spectacle-wearing birds relied upon their unaltered auditory map and turned their heads to the same degree as they normally would without the goggles. But after six to eight weeks, their brains recalibrated, adjusting to the visual distortion caused by the glasses. Now, when the birds heard the noise, they swiveled their heads even farther than before, because the new visual map had apparently instructed the auditory map to shift as well. While experimenting with the prismatic lenses, Knudsen again found that young birds had an easier time compensating for the distorted visual cues than older birds did. When they wore prisms that shifted their field of view 23 degrees, juvenile owls adjusted to the distorted cues after just a few months. By comparison, adult birds achieved at most only a quarter of the adjustments that their younger counterparts did. Electrodes recorded neural activity in two different areas of the older birds’ brains—indicating that their maps weren’t realigning. The difficulty adult birds face when trying to adapt to the lenses fits with the current understanding of critical periods. Later in life, the brain is better at recalling learned information, rather than learning itself. “Your brain becomes more committed when you are older,” explains Knudsen. “There is a tremendous advantage in reliability.” But Brie Linkenhoker, a graduate student in Knudsen’s lab, wondered if there wasn’t still some latent malleability in the adult birds brains, so she decided to try teaching these older owls in small steps. Rather than shifting their vision a full 23 degrees, Linkenhoker started the owls with shifts of 6 degrees, then 11 degrees, and finally 17 degrees. To her surprise, the incremental learning made a big difference. Although the mature birds still didn’t compensate by correcting their mental maps as much as the juveniles did, they were far more malleable than when faced with one big visual shift. Linkenhoker’s experiments offer a new research regime for those studying how adult brains learn. The results also suggest that whatever the instructive signal from the visual system is, it operates over a small range, yet could still be exploited. “We have to ask, what is the relative practice step for [learning in] a given system, and then repeat that step,” she says. “The idea now is repeat, repeat, repeat.” Within the barn owl system, the small step was easy to figure out: shorter, incremental shifts in the visual field. But Knudsen says that if we can figure out the small steps for absorbing other kinds of information, adult brains might be able to learn much more than scientists previously thought. He hopes that someday, that kind of knowledge could help improve the recovery of patients with brain injuries. Sascha du Lac, a former grad student of Knudsen’s who now works at the Salk Institute in La Jolla, California, agrees. “The dogma is that synaptic connections between neurons change,” says du Lac, “But the change may be in the firing of the neurons.” If that’s the case, the neural architecture may still be present. If scientists could find the right stimulus to wake the latent neurons, it would be possible to revive forgotten or unused connections. Additional experiments by Knudsen suggest that in barn owls, once the neural architecture is laid down, it persists. He’s recently homed in on a type of a nerve cell that has already been implicated in learning processes in other parts of the brain. These cells play an important role when the birds are actively learning a new mental map, and seem to suppress the old map when it is no longer useful. Studies of how infants learn languages support the notion that the brain’s learning capacity depends not only on the amount of new information at hand, but also on how it’s packaged. Linguist Tobey Nelson and her colleagues at the University of Washington in Seattle recently conducted a study in which they exposed two groups of 9-month-old infants to Mandarin Chinese. One group of babies listened to a CD of someone speaking Mandarin, while the other group heard the language spoken in person by four adults. Both groups were then tested for their ability to distinguish between two Mandarin sounds. The children who listened to the CD were no better at recognizing the sounds than a control group of kids who’d only listened to English. But the infants who heard the language live, and from different speakers, fared significantly better on the test. “Even at very young ages, we are aware of a connection between visual and auditory information,” says Nelson, who works at the university’s Center for Mind, Brain and Learning. “What you are doing while hearing—the social interaction, making eye contact—it makes a difference.” Nelson’s work suggests that the brain pays attention to a lot more than just sound when learning language. “The brain really is quite plastic,” she says, “But we have to figure out how to package the information, to tailor the training in the most effective way.” Preliminary data from the latest work in Knudsen’s lab lends further support to that idea. Prism-wearing owls seem to adjust their mental maps more quickly if they have to catch their dinner, rather than being fed—in other words, when it really matters to their survival. “If you care about something, you learn more than if you don’t care about it,” he says. So in addition to packaging new information in small bites, the context within which the learning occurs seems to be critical. Says Knudsen, “It’s a combination of making the information graspable, and the amount of arousal—the amount of attention you engage the animal with—that will determine how much information it can gather.” ![]() |
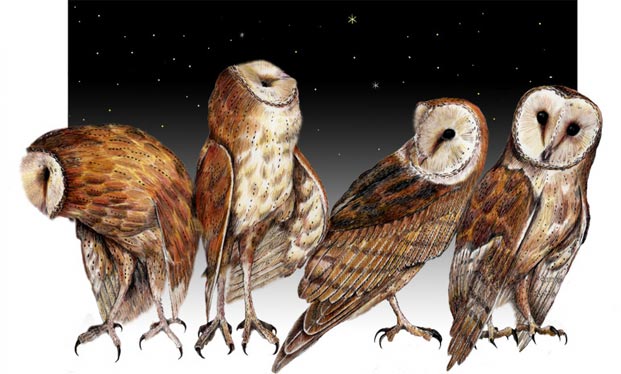