Is there life on Mars? The question can provoke strange reactions. For some, it calls to mind visions of little green men, fang-toothed and nefarious, coveting the cool water and lush forests of Earth. Others recall Orson Welles famous 1938 "War of the Worlds" radio broadcast, when an announcer warned stunned listeners that "the vanguard of an invading army from the planet Mars" had landed in New Jersey.
When UC Berkeley chemist Richard Mathies wonders whether theres life on Mars, however, he thinks of the blazing red and orange industrial salt ponds in the South San Francisco Bay. These thousands of acres of salt-producing evaporation ponds get their vivid colors from clouds of bacteria that thrive in extremely saline conditions fatal to most other living things. Mathies believes that if life ever existed on Mars, it probably looked a lot like these hardy bacteria.
But we know that no matter how tough and resilient a terrestrial life form is, it needs water to survive. And were all but certain that water once flowed on Mars. NASAs Mars rovers, Spirit and Opportunity, have gathered evidence that water once flowed over the surface of our red neighbor. They found sulfur-rich "evaporites" minerals that remain when a brackish, acidic body of water dries up. "There are clearly areas on Mars where there was once a bunch of water that evaporated and left all this stuff," says Mathies. "And on Earth, the last things that live before the whole thing dries up are these little bugs." Its only logical, Mathies argues, to begin the search for life on Mars by looking for traces of hard-boiled, tenacious microbes.
Before anyone can search for signs of life, theyll need instruments capable of finding them. Mathies and his team at UC Berkeley are developing the Mars Organic Analyzer, an exceedingly sensitive device that can detect tiny traces of amino acids the protein building blocks essential to life.
The analyzer is tiny; the main chamber is a wafer-thin "lab on a chip" no bigger than a beer coaster, and the entire unit is about the size of a shoebox. This miniature size is essential. If all goes according to plan, it will be packed aboard the European Space Agencys ExoMars rover in 2011, along with a suite of similarly compact exploration tools.
Before ExoMars lifts off, Mathies and his team will have to make the next analyzer prototype even smaller. They must also construct it out of more durable materials, to ensure it can survive the subzero temperatures and low pressures of Mars. Each new incarnation will require exhaustive testing to prove that the device is functional and rugged enough for a costly space mission.
But for Mathies, the possibility of finding life on Mars makes all the effort worthwhile. "The instruments that have been to Mars so far are inadequate for answering the kinds of questions we want to pursue," he says. "But our system is up to 10,000 times more sensitive. The technology is mind boggling."
The Big Zinger
The search for life on Mars hasnt always been greeted with such open arms. Nevertheless, Chris McKay of NASAs Ames Research Center in Mountain View, California, believes the scientific community is ready to ease its skepticism. "Theres been a remarkable change in attitude over the last ten years. Evidence for water on Mars is partly responsible," McKay says. "Now its everybodys favorite question." Even so, deciding what evidence to pursue in the search for life is still contentious.
Some suggest looking for DNA, some for its sister molecule RNA; others say fossil evidence of whole microbes would make more sense. The analysis of a Martian meteorite found in Antarctica which some argue teems with such fossils revived interest in Martian life in the mid-1990s. But Mathies is convinced that the search should look for more basic clues.
"It turns out that amino acids make up over 90 percent of the physical dry weight of bacteria," Mathies says. Furthermore, he argues that amino acids can survive intact for tens of thousands of years, resisting degradation even in a Mars-like environment. Looking for amino acids is the obvious choice, he says.
Jack Farmer, an astrobiologist from Arizona State University, isnt so sure. "These compounds are typically short lived in a geological environment, so I think it may be a long shot to find them in rocks," he says. On the other hand, "we might be able to find amino acids or proteins indicative of biology preserved in ice."
Amino acids dont need a living organism to make them. Chemists Stanley Miller and Harold Urey demonstrated as much in their famous "primordial soup" experiment in 1953. They mixed together a batch of ammonia, methane, hydrogen, and water in a closed, sterile container, and then zapped it with an electric current for a week. What resulted was a sauce of organic compounds, including amino acids and not one single cell was asked to participate.
Evidence like this suggests scientists need a way to distinguish spontaneously occurring amino acids from those produced by living cells. Mathies says the key to solving the problem the "big zinger," as he calls it is a structural property known as chirality. Amino acids with the same molecular formula exist in two different physical forms. Like a pair of hands, each form is a mirror image of the other. Appropriately, chirality is also called "handedness," and chiral forms are called either "left-handed" or "right-handed."
A batch of amino acids made without any help from living cells will contain a nearly equal mix of left-handed and right-handed forms. But in samples created by life, one form will dominate. On earth, every amino acid in the proteins that make up our cells is left-handed. But thats no reason to assume life elsewhere in the solar system will obey the same rules.
"The idea is to make your hypotheses as general as possible," Mathies says. "You can look for nucleic acids, but I think thats way too Earth-centric. Youre making the assumption that life on Mars basically evolved with the same handedness, which strikes me as very restrictive."
On this point, Farmer agrees. "When you go to another planet like Mars, you have to think about it differently than people have in the past," he says. "We dont really understand where life came from on our own planet. Theres a lot of complexity there that has not been filled in."
And so the analyzer will look for hardy, long-lived amino acids on Mars to cast as wide a net as possible. If it finds any, it will examine their chirality to determine whether the suspects are microbial remnants. A random mixture of amino acids without a dominant handedness could just be dribble from the cosmic soup ladle.
An Organic Handshake
Before chiral analysis can happen, the first task is to identify amino acids in a sample. Enter Jeffrey Bada of the Scripps Institution of Oceanography in La Jolla, California. He had already designed a device called the Mars Organic Detector when he and Mathies started collaborating. But his detector could only tell part of the story.
"The instrument, as conceived, would only detect the presence of organic compounds," Bada says. "Then I started asking if we could take the concept a step further and analyze the structure, the handedness of amino acids." It seemed only natural that Mathies and Bada would team up.
NASA had selected the detector for a Mars mission scheduled for 2003. But then the Mars Polar Lander crashed in 1999, and NASA scrapped the 2003 mission. Undeterred, Bada continued work on the detector. He and Mathies co-authored a paper in the journal Analytical Chemistry demonstrating the first version of the analyzer unit in 1999. "After that, we received some pretty robust support from NASA to continue the work," Bada says.
The idea is to mate the detector with the analyzer to make an integrated machine. In this system, the detector first isolates any organic material from a soil sample. It is then mixed with a special fluorescent tagging dye called fluorescamine that lights up only when it touches amino acids. If the detector succeeds, the sample enters the analyzer to determine its chirality. The "lab on a chip" portion of the analyzer has tiny channels filled with a compound called cyclodextrin. This stuff is attracted to amino acids, but it sticks to only one of the chiral forms. By exploiting this characteristic, the analyzer can sort one form from the other.
To envision the process, imagine you are a politician walking down a runway and shaking hands with as many voters as you can. "If you hold out your right hand, and all the people hold out their right hands, you can shake with every person, and it takes some time to make it down the runway," Mathies says. "But if instead you hold out your left hand, the hands dont meet, and you walk down the runway at a quicker rate."
In the analyzer, the cyclodextrin molecules are the people in the crowd, and the amino acids are the politicians. By measuring the rate at which the amino acids move down the channel in the chip, the analyzer can measure the ratio of right-handed to left-handed forms.
"In terms of planetary exploration, this instrument is going after a particular answer that nobody could address before," says Alison Skelley, a doctoral student in Mathies lab and designer of the MOAs chip component.
A Single Drop in a Swimming Pool
The MOA may find a preponderance of left-handed amino acids, which could point toward life. But this finding would come with a major caveat: It might be hard to ensure that the result is not simply due to hitchhiking organisms brought from Earth.
Farmer believes the device shows much promise in evaluating such "forward contamination." "When we go to Mars or other planetary environments, were going to have to be pretty bulletproof about detecting ourselves," he explains. "Its impossible to completely sterilize a spacecraft. My sense is that the early applications of these kinds of instruments will probably be in the detection of forward contamination."
Alternately, the MOA could find a blend of both chiral forms, known to chemists as a "racemic" mixture. This result would suggest a nonliving source, especially if the ratio is close to 50/50.
Mathies would be particularly excited to find a majority of right-handed amino acids. "That would be a fabulous result. It would tell us its possible to evolve life with opposite handedness than life as we know it. And, we wouldnt have to worry about contamination."
"Finding life that developed on a fundamentally different pathway would change our whole perspective about what life is," Farmer notes. "On the other hand, if we found life that fit right into our own tree, we would have to entertain the possibility that were all Martians, or Martians are us. Either way, it has huge philosophical implications."
But Skelley sees no reason to give up even if the MOA finds a racemic, or balanced, mixture. "It could mean there may have been life, but it was there many years ago," she says. She explains that on Earth, a sample of left-handed amino acids from cells will convert to a racemic mixture over time. "You can actually use this as a way of dating how long ago it died off, but you have to know the conditions it was in." Temperature, pressure, and acidity can all affect how quickly amino acids flip to a balanced mixture, Skelley notes. So if samples display an unevenly racemic mixture, say 70/30, this could suggest life may have existed in the past. This would naturally open up a whole new set of questions to answer.
Mathies, Bada, and Skelley are confident that if amino acids exist on Mars, the MOA will find them. The system is so sensitive, it can potentially detect traces as low as 10 parts per trillion. "Take a reasonably good sized swimming pool," Mathies explains. "Now put one drop of your analyte in that pool and stir it up. Thats the level of sensitivity we have with the MOA. We can detect that one drop in 30,000 gallons."
Bada sums it up more succinctly: "Were looking for a needle in a haystack."
Mars on Earth
Before the MOA goes anywhere, it must endure punishing trials to make sure it can withstand conditions on the frozen surface of Mars. Like all Mars-bound equipment, the device will suffer the desolate wasteland of Chiles Atacama Desert the driest, most Mars-like environment on Earth. As Mathies puts it, "If you cant detect life in the Atacama, you have no business going to Mars."
The first round of tests in the Atacama took place in early 2004. Since the analyzer wasnt yet ready for field testing, the researchers collected samples prepared by the detector in the Atacama and brought them back to California to test the analyzer. These tests detected amino acids at levels as low as 10 parts per billion. But the chirality analysis was stymied by unusually high salt concentrations in the samples.
This spring, Skelley will take the entire detector/analyzer unit on its first field trip to the Atacama. She plans to dig deeper into the soil, partly to escape salty deposits at the surface. But she also wants to explore the prospects of detecting amino acids up to a meter below the sun-blasted surface.
Skelley, Mathies, and Bada agree that both in the Atacama and on Mars, digging this deep will be crucial. At the surface, harsh conditions such as temperature fluctuations and cosmic radiation threaten the long-term survival of amino acids. But deeper down, the overlying soil provides a protective shield. "If we can demonstrate this approach in the Atacama, it means that not only can we successfully detect things, but it also gives a very reasonable method for performing the experiment on Mars," Skelley says.
Skelley tested the analyzer in the Panoche Valley east of Salinas, California, in early 2004. The team chose the site for its abundance of sulfur-rich jarosite, a mineral that forms only in the presence of water. The choice was serendipitous just before the field tests took place, the Opportunity rover had found deposits of jarosite on Mars. The MOA found amino acid traces as minute as 70 parts per trillion within reach of the 10 parts per trillion goal in the Panoche jarosite.
In finding evidence for water, the Mars rovers also have identified promising sites to begin searching for traces of life. "The presence of sulfate minerals on Mars could make the search for organic compounds much easier," explains Bada. Life-seeking missions may only have to look through a small handful of hay rather than an entire haystack for that elusive needle. "And sulfates are also a pretty good shield for radiation, so organic matter near the surface may be better preserved," Bada adds.
No matter how harsh the desert environments are on Earth, the frigid temperatures and low pressures of the Martian surface cannot be reproduced in a natural setting on Earth. To do this, the team will test the detector/analyzer unit in the "Mars Chamber" at NASAs Jet Propulsion Laboratory in Pasadena. Conditions in this simulation room can plummet to below -100° C and 0.5 % of Earths pressure.
But the current incarnation of the analyzer is made of relatively inexpensive and fragile components. While its ideally suited to earthbound proof-of-principle experiments, it wont stand up to the punishment the Mars Chamber can dish out. To prepare for these tests and the challenges beyond, Skelley will construct a new prototype of tougher, spaceflight-capable stuff. She and the team are in the process of testing and choosing appropriate materials.
A Change in Plans
The MOA was originally under consideration for NASAs Mars Science Laboratory mission in 2009. But just recently, the team learned that NASA decided to pass. "The device was rated extremely favorably with regard to science, but they believed it was not yet technically ready to fly," says Bada. "They said its a beautiful instrument for future missions; its just not ready for this one."
McKay, although not directly involved with the MSL decision, provides some insight. "The selections for these missions are always hard. There are always more good instruments than there are funds or resources to support them," he explains. "I dont think its necessarily the case that the instrument wasnt suitable. We wanted more evidence that there really is organic material on Mars before we send an instrument specifically to analyze for it."
Although disappointed with NASAs decision, Mathies sees a bright flip side. ESA had struggled to give its mission a unique identity. The exclusion of the analyzer from the NASA probe will help make this distinction clear, he explains. Moreover, ExoMars is a better fit for the scientific goals set by the MOA team. "The NASA lander wont be capable of digging deeply into the Martian crust, and so is unlikely to get at the molecules were interested in. But ExoMars will be digging a half-meter to a meter down," Mathies says.
The deeper we dig in the search for life, the more surprises we are likely
to find. On Earth, weve found something living in nearly every
cracked boulder weve drilled into, every boiling hot spring
weve drawn from, and every dried salt pond weve sifted through.
If there are microbes lurking elsewhere in the solar system, well
need tools like the MOA to shake hands with their tiny molecular
ambassadors. What we find just might force us to rewrite all of our biology
textbooks.
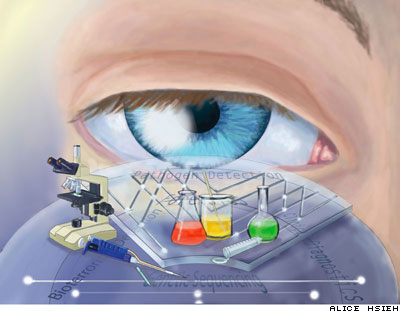
Laboratory as Carry-on Luggage
Lab-on-a-chip technology, like that used in the Mars Organic Analyzer (MOA), is about to shrink the scale of biochemistry research. Soon, these chips and their miniature fluid circuits might perform experiments that would take a roomful of bulky lab equipment today. They will also use smaller amounts of expensive chemicals and irreplaceable samples in the process.
Mathies believes the technology can help with just about anything scientists do, from rooting out bioterrorists and criminals to sequencing genes and diagnosing diseases. Ideally theyll be able to do it quicker, and with less energy, too.
Chemists have gone through the test-tube era, Mathies says. Test tubes are good for measuring in milliliters, each of which is about the size of a small marble. But today chemists use things called microcentrifuge tubes and microtiter dishes, which measure in microliters. Each microliter is about the size of a pinhead, or about a thousand times smaller than the marble.
But Mathies wants to go even smaller. The amount of molecules it takes to do a reaction is not very much; it really only takes nanoliters. Once again, this means a volume a thousand times tinier smaller than the sharp end of the same pin.
Much like computer chips use microelectronic circuits to carry digital signals, labs-on-a-chip use microfluidic circuits to move and mix liquids. Even the appearance of a lab-on-a-chip is reminiscent of its electronic cousins: the shape of the liquid channels looks like an etched silicon circuit panel, and wire-like tubing is attached to the edges of the chip.
For Mathies, its a good comparison. The big vision is really a chemical microprocessor, he says. Since electronic microprocessors have allowed computers to shrink from enormous, room-sized machines down to laptop-sized notebooks, Mathies believes microfluidic chips might soon make lab rooms full of messy tubing, glassware, and machines obsolete.
In a computer, the chip is only the central part of a larger system. Its the same for labs-on-a-chip. In the MOA, for example, optics and electronics in the shoebox-sized unit are all vital to get the job done. Much like our cell phones and digital cameras keep getting smaller, these electronic units will also.
Labs-on-a-chip may also become the heart of portable analyzer gadgets. For example, a forensics investigator may be able to bring a small device to a crime scene and process DNA samples on the spot, reducing the chance of a sample mix-up. Toxicologists could analyze a potentially contaminated water supply at the source, enabling them to get the word out quickly.
Were not going to be manipulating macroscopic amounts of
stuff on a lab bench anymore, Mathies says. This technology
is going to transform how and where we do chemistry and biology in the next
few decades.
ABOUT THE WRITER
Matthew Early Wright
B.S. (biology), University of New Mexico
M.S. (biology), Arizona State University
ABOUT THE ILLUSTRATORS
In college, Emily Cooper was told by her student advisor, "You can do what you like, Emily, but unless you're doing art, you won't be satisfied." She resisted this prophecy for a long time, gathering other skills through teaching and helping build a software company. Still, she was always searching for a profession that would provide both constant opportunities for learning, and the chance to create a legacy she could be proud of. Science Illustration fit the bill perfectly. Now, freshly graduated from the UCSC-Extension Science Illustration program and looking forward to an internship at Scientific American magazine, she thinks she’s really ready to make this new career happen. And we’ll see: perhaps her old advisor’s prophecy will be fulfilled!
Alice Hsieh graduated from UC Berkeley in 2002 with a major in Integrative Biology and a minor in Art. In the two years before she applied to the Science Illustration Program at UCSC, she has done everything from trapping rats in the Arizona desert and taking care of arthropods at the San Francisco Insect Zoo to working for a small business selling plastic plants and trees. She hopes she can put her desk job behind her and dreams of heading through some exotic local with a field researcher and illustrating what she sees. She also hopes that she will have the chance to illustrate in an educational context as well.