
By GRETCHEN VOGEL
Illustration by DAVID FIERSTEIN
HOW MUCH DOES THE UNIVERSE WEIGH?
In the science fiction series, The Hitchhiker's Guide to the Galaxy, by Douglas Adams, the answer to the question of "Life the Universe and Everything" is 42. In astrophysics and cosmology, researchers and theorists are also searching for numbers. These cosmological constants will help them understand how our universe formed, how it behaves now, and how it might end. One of the cosmological constants is the mass of all the atoms in the universe. Thanks to the Keck telescope, the largest light-collecting telescope on earth, and an instrument connected to it called the High Resolution Echelle Spectrograph or HIRES, scientists are much closer to knowing the number.
Of course, there is no scale or balance scientists can use to weigh the universe. But scientists think they can determine the figure indirectly by looking at which elements formed at the very beginning of time. HIRES is letting them do this.
WHY THE MISSING MATTER MATTERS
One reason scientist want to know the mass of the universe is that it seems there is matter missing from space. Astrophysicists have calculated that their telescopes can detect only about one-tenth of the matter in the universe. They call the unseen 90 percent "dark matter," and they have been trying to find it for nearly two decades. But they aren't sure what they are looking for, which makes the search more difficult. HIRES can help them figure out what to look for.
The dark matter might come in two "flavors." It could be regular matter we just can't see because it doesn't give off light or other radiation, or it could be bizarre particles familiar only to high-energy physicists and detected only in their atom-smashing particle accelerators. Scientists believe they can determine how much regular matter (also called baryonic matter) there is in the universe. If they can do this, then they can compare that number to the amount of matter we can see. The difference must be the "missing" baryonic dark matter.
THE PING PONG UNIVERSE
The indirect scale physicists use to measure the mass of the universe is based on the theory of the big bang. Physicists think they know how the first building blocks of matter - protons and neutrons - collided in the first moments after the big bang to create the first elements. By measuring the relative amounts of those different elements, scientists can calculate the original density of matter and the present-day mass.
It might be easier to think of the early universe as a room full of floating ping pong balls. The balls have Velcro on them, and they stick together when they collide. One (indirect) way to determine how many balls are in the room is to measure how many are stuck together in pairs and trios at the end of, say, three minutes. If the room is more crowded, more ping pong balls will collide and stick together. Astrophysicists use the same principle to determine the mass of the universe.
Deuterium, a heavier version of hydrogen, is one of the elements created by the colliding particles shortly after the big bang. The creation of deuterium was especially sensitive to that original density of particles. Therefore, if scientists can pin down how much deuterium was created in the moments after the big bang, then they can calculate how much element-forming matter was there altogether.
THE BIG BANG
According to the Big Bang theory of cosmology, the universe was once an incredibly small and dense point. That point exploded into the primary particles of matter: electrons, protons and neutrons, as well as more exotic particles. In the first few minutes after this explosion, intense heat and pressure forced the newly formed particles into atomic nuclei - the cosmic precursors of atoms. Only the simplest nuclei had time to form, however, before the universe got too large and too cool to force the particles together. There was time to form only hydrogen (the simplest nucleus), helium (the second smallest), and a small amount of lithium and beryllium, the atoms just larger than helium.
A hydrogen nucleus contains two particles, so it requires only one collision to form. A deuterium nucleus contains three particles, so it requires two collisions. The simplest helium nucleus contains four particles, so it requires three collisions. The density of the early universe - how tightly the particles were packed together - determined how much of each element formed. Tightly packed particles undergo more collisions than spread-out particles, so if the universe was very dense to begin with, more deuterium would have been converted to helium in those first three minutes. If the universe was less dense, more particles would have stayed in relatively unstable deuterium nuclei. Therefore, by measuring the amount of deuterium present compared to the amount of hydrogen present, scientists can figure out how dense the particles were.
Scientists have been searching for the correct deuterium ratio for more than 20 years. But until last year, the generally accepted values were calculated from observations of gas and dust in our galaxy. Our galaxy is made largely of exploded stars. Deuterium is a fragile element, and the nuclear reactions in stars readily destroy it, so scientists had to use complicated and imprecise theories about star formation to estimate how much of the element has been destroyed during cycles of star birth and death. Astronomers think a better measurement can be taken outside our galaxy. And for the first time such a measurement is possible with the Keck telescope and with HIRES.
KECK AND HIRES LOOK BACK IN TIME
The scientists who use Keck call it a "big light bucket." It sits on top of a snow-capped, extinct volcano on the Hawaiian island Mauna Kea. Its main light-collecting surface is 10 meters in diameter and allows Keck to collect more than six times as much light as any other telescope. This allows scientists to study very dim, very distant objects.
The High Resolution Echelle Spectrograph is an instrument that allows scientists to analyze this light. Just as a prism sorts visible light into its rainbow of colors, the HIRES instrument sorts the starlight into a spectrum. Different light sources give off different colors of light, and scientists can read the spectrum to determine what created the light.

HIRES sorts light from Keck into separate wavelengths so scientists can analyze it.
(1) Light from Keck enters here and travels to the "collimator" (2).
(2) The collimator is a mirror that straightens the light beam on its way to the diffraction grating (3).
(3) The world's largest echelle diffraction grating acts like a prism to divide the light beam into separate wavelengths, or colors. The light then travels to another diffraction grating called the cross disperser (4).
(4) The cross disperser splits the light again into even finer wavelengths. The light then travels through two correction lenses (6), which aim it at the mirror (7).
(7) The mirror reflects the separated light onto the electronic sensor (8).
Because light takes time to travel from one point in space to another, light from distant stars reveals what the universe was like long ago. Quasars are some of the oldest objects that optical telescopes can detect. Billions of years ago, shortly after a quasar emitted the light we see today, the light passed through a series of gas clouds. Astrophysicists think the light passed through the clouds before any galaxies or stars had formed. Two teams have used HIRES to look for the fingerprint of deuterium in the absorption patterns of quasars. They have found quite different answers.
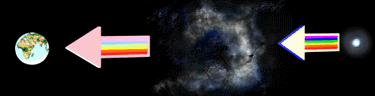
Along its journey to earth, the light from a quasar travels through clouds of gas and dust. These absorb some of the light, leaving shadows, or "absorption lines" in the spectrum. Astrophysicists can tell from the absorption lines what is in the gas clouds.
WHAT THE ASTROPHYSICISTS HAVE FOUND
In 1994, Antoinette Songaila, Esther Hu and Lennox Cowie, all astrophysicists at the University of Hawaii's Institute for Astronomy, analyzed the light from a quasar and found evidence for a ratio of deuterium to hydrogen several times higher than the value calculated from observations of deuterium in our galaxy.
But in May 1996, David Tytler, Xiao-Ming Fan and Scott Burles of the University of California, San Diego published evidence for a deuterium-to-hydrogen ratio ten times lower than that of Songaila's team.
A higher deuterium measurement corresponds to a lower density of matter. If Songaila's numbers are right, there is less normal, or "baryonic," matter in the universe than scientists had thought. In fact, Songaila says, if you use the deuterium ratio her team found, the amount of regular matter in the universe "needn't be any larger than the amount you can see in the universe simply because it's lit up." Which means that 90 percent of the universe might be made up of non-baryonic, exotic particles.
But Songaila readily admits that their numbers are not above question. The area of the spectrum she and her colleagues study is in what astrophysicists call the Lyman-alpha forest. It is a region of absorption lines so thick that they overlap onto one another and hide behind one another like trees in a forest. Sorting out individual lines of hydrogen and deuterium is not unlike picking out which seedling in a thicket came from which tree. The final answer contains an unavoidable amount of uncertainty.
Tytler, on the other hand, is confident that his number is correct. The data are too different, he says, for both numbers to be right. He is confident in the systematic approach he and his team used, he says. They analyzed dozens of quasars at the smaller Lick Observatory to look for clear deuterium signals, and they focused in on just a few of their most promising candidates at Keck. And they have found their deuterium ratio in two quasars in two different parts of the sky.
If Tytler's numbers are correct, the dark matter story is a little bit different. His lower deuterium ratio indicates that there is much more regular matter out there than astronomers can see. "If you add up everything we've ever seen, that accounts for only 10 percent of this number," he says, which means that much of the dark matter astronomers are looking for may be regular, baryonic matter. It doesn't rule out the possibility of exotic particles, but it means there may not be as many as Songaila's results predict.
THE DEBATE CONTINUES
Songaila is not concerned, however. "The safest thing to say at this point is that our measurements bracket the possibilities," says Songaila. "We found a high value, and that's probably an upper limit. I would say it is reasonable to classify (Tytler's) as a lower limit." And she freely admits that more studies are necessary. It is not easy to find quasars with the right characteristics to study, however. Songaila and her colleagues have looked at five or six other quasars, but haven't been able to find deuterium in the forest.
Wallace Sargent, an astrophysicist at the California Institute of Technology who also uses HIRES to study the absorption patterns in quasar light, thinks Songaila and her colleagues made too many leaps of faith in their interpretations of the lines. He says they are foolish to look in the Lyman-alpha forest for the deuterium measurement. "If they think they can see a little blip on their spectrum and can call it deuterium, they are crazy," he says. "It's a case of fools rushing in where pundits fear to tread."
BUT ALL AGREE ON ONE THING
Despite the controversy over the data, all who have used HIRES agree that it is a near-flawless instrument. "HIRES makes a tremendous difference in our ability to look at quasar absorption spectra," Sargent says. "It's a beautiful piece of machinery."
Tytler goes even further. "You couldn't measure the deuterium without it," he says. "It was in a sense designed to do exactly this sort of thing, so it's very good at it. The thing about HIRES is that it works. It works reliably, and it does what it's supposed to do."
Songaila agrees. "HIRES is so well put together and so beautifully engineered it's almost boring," she says. "Things don't go wrong. You just plug in, tell it what to do and it just goes off and does it. There is no drama."
She predicts that Keck and HIRES will help determine a solid value for the deuterium ratio within the next few years. "If HIRES can't do it, I don't quite see what will," she says. "We simply have to find more systems, and that's simply a matter of plugging away and being lucky."
|