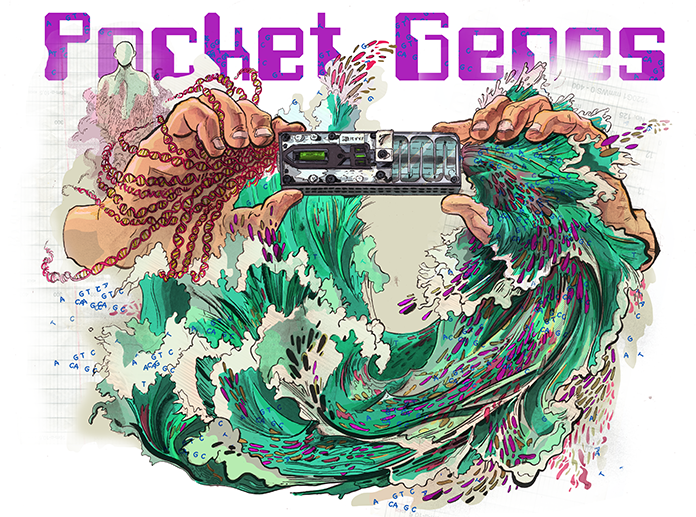
|
Illustration: Jay Rasgorshek |
A miniature DNA sequencer began 27 years ago with a quick sketch in a car. Laurel Hamers spells out the tale. Illustrated by Jay Rasgorshek.
David Deamer was driving through Oregon when he swerved his car to the side of the highway. He dug out a steno pad and a red pen—“the only pen I had”—and hastily recorded the thought that had come to him moments before. What if, he wondered, you could detect the genetic information encoded in a strand of DNA by threading it through a microscopic hole in a cell membrane? By measuring tiny changes in electrical current as the strand passed through, one might be able to read the blueprint for life it contained. He dated the diagram June 25, 1989.
Deamer, now a research professor in biomolecular engineering at UC Santa Cruz, still has that notebook. His doodles sparked a quarter-century of research on the membrane holes known as nanopores. The intensive work—and $200 million in investments—paid off in 2014, when a British company called Oxford Nanopore released a pocket-sized device that uses the technology Deamer envisioned. The “MinION” translates DNA’s molecular message into one scientists can understand.
Deamer began investigating his idea as teams were racing to sequence, or read, the entire human genome for the first time. In 2001, researchers with the Human Genome Project unveiled our instruction manual: a complete sequence of about three billion DNA subunits, called bases, encoding the roughly 20,000 genes that shape our identities. (A team at UCSC published the first such sequence online.) But most mainstream DNA sequencers remained hulking machines, costing upwards of $100,000 and weighing nearly as much as a Mini Cooper. In contrast, the MinION sells for less than $1,000 and is smaller than an iPhone.
Eminent scientists told Deamer and his colleagues their vision would never work, and lawsuits almost derailed the project. But today, the MinION is transforming DNA sequencing by taking the technology into the field, where it helps scientists track diseases and identify species within hours. It calls to mind the morphing of computers 30 years ago from room-sized behemoths into desktop devices that anyone could own. Deamer’s chief collaborator, UCSC’s Mark Akeson, is succinct about what the MinION represents: “A democratization of sequencing.”
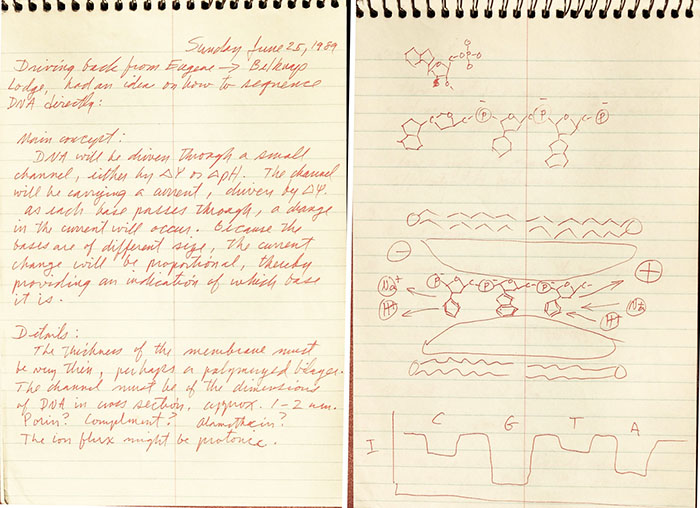 |
Image courtesy of David Deamer |
Deamer’s notes on his nanopore sequencing idea, drawn during a car trip in 1989. |
Making the MinION
The MinION is a sleek black box the size and weight of a flip phone. A lid swings open to reveal a glimpse of its inner workings. When it’s hooked up to a laptop, rainbow lights blink down the side and colorful lines representing different sizes and numbers of DNA molecules march up the computer screen—the only sign that sequencing is in progress. The real action happens on a nanoscopic level, where hundreds of pores work in much the same way that Deamer, now 77, dreamed of in his sketch.
Deamer’s office is decorated with testaments to the journey, like a 3D printed model of the first nanopore through which he threaded DNA. Tall and lanky with a finely trimmed white beard, he’s quick to jump up and draw diagrams. Even the simplest question is an opportunity for him to weave a tale. He punctuates each thoughtful, clearly enunciated sentence with a flourish of detail and an inviting wave of a hand. (“You’re the first person who’s heard this story start to finish,” he told me, leaning back in his leather chair.)
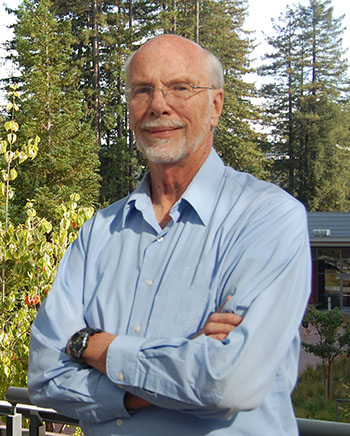 |
Photo: Miten Jain |
David Deamer on the terrace of UCSC’s main engineering building. |
|
But Deamer didn’t set out to sequence DNA. For decades, he’s been captivated by the origins of life: how, long ago, molecules drifting in a thin soup of chemicals assembled into primitive cells that could make copies of themselves. He’s visited sulfurous pools in Siberia and pulled apart meteorites in a quest to understand what triggered that unlikely transition. At the time of his roadside revelation, he was studying how membranes might have come together on the young Earth to create those earliest cells. As part of his work, he was trying to pass the four bases of DNA and its sister molecule, RNA, through the membrane of an artificial cell. “But nobody had found a big enough pore,” he recalls. Still, he reasoned, if a pore could accommodate the four bases, why not a full DNA molecule?
DNA’s bases—adenine (A), thymine (T), guanine (G) and cytosine (C)—link together into sequences that guide cells to create proteins, which are essential to all known life. Pairs of these units form the rungs of the curving ladder-like DNA structure, the classic double helix. Large molecules like DNA can’t move freely through a cell membrane, but certain proteins embedded into the membrane can act as gatekeepers. Under the right circumstances, they’ll let specific molecules enter or leave the cell.
There’s usually an electrical charge difference across a cellular membrane. One side is more negative, the other more positive. It’s enough of a difference—about one-tenth of a volt—to push electrically charged ions in and out of the cell. Nerve cells use this process to transmit signals.
Since DNA is negatively charged, a voltage might pull it through a membrane channel, Deamer thought. Usually, DNA stays in a cell’s nucleus. But inside an artificial membrane, DNA extracted from a cell would move to the positively charged side if a hole was wide enough. Each chunky base moving through might briefly block the channel, temporarily changing its current of ions. And because A, T, G, and C are chemically and structurally distinct, each might create its own electrical signature. Those blips could be a new way to map the sequence of DNA—and its genetic information.
The idea lingered in Deamer’s mind for two years before he ran it past his former postdoctoral advisor, Harvard University biologist Daniel Branton. “I thought that was a terrific notion,” says Branton today. They filed for a patent.
But to test the idea, they first needed to find a pore large enough for DNA to navigate. That inspiration came from a conference talk given by researcher John Kasianowicz about a membrane protein called hemolysin, which makes red blood cells swell and burst. Deamer and Branton suspected the tiny hole in the middle of the protein (20,000 times narrower than a human hair) might allow a single strand of DNA to wriggle through.
Deamer visited Kasianowicz in 1993 at the National Institute of Standards and Technology in Gaithersburg, Maryland. The scientists jammed a hemolysin pore into an artificial membrane and recorded the electric current moving through it. When they added strands of RNA, the signal changed right away. As he recalls this scene, Deamer makes gunshot noises to simulate each single molecule being pulled through the pore in a few milliseconds.
When he returned a few months later with Branton, they added an enzyme to chop the RNA into small bits. As the enzyme gradually destroyed the RNA, the signal disappeared. There was nothing left to read. “So we said to ourselves, this is it,” says Deamer. “But then we had to convince other people.”
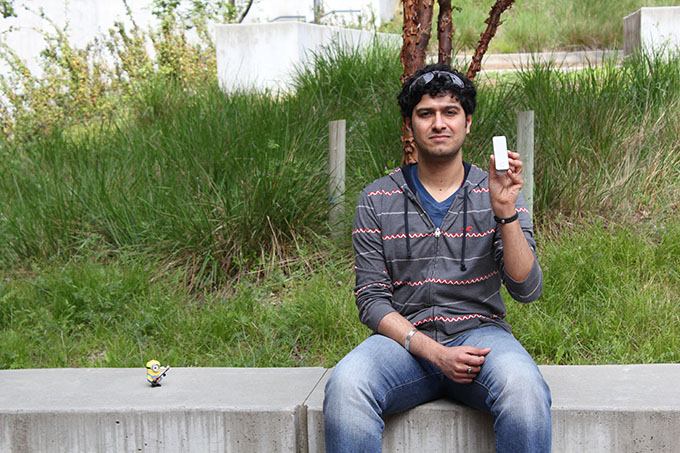 |
Photo: Laurel Hamers |
UC Santa Cruz graduate student Miten Jain holds a MinION—with a guitar-playing Minion action figure looking on. |
|
From sketch to solution
Winning supporters was challenging at first. “One [skeptic] even went on record to say, ‘This is like cold fusion. This is impossible,’” says Deamer.
To sequence DNA, scientists must unwind its double-stranded helix and read one strand at a time. The team soon showed that single-stranded DNA sparked a change in voltage as it slid through the pore, but double-stranded DNA didn’t affect the current. It was too wide to squeeze through. That meant the signal they were recording indeed came from a single strand, they concluded.
Those results, published in 1996, “proved to me that it was not totally fantasy,” says Mark Akeson, a former postdoctoral associate of Deamer who worked at the National Institutes of Health. So when Deamer offered him a temporary position at UCSC to work on nanopore sequencing, Akeson left his stable job and moved his family cross-country to Santa Cruz. (He’s still there; he bought a house just up the hill from Deamer.)
Deep skepticism persisted elsewhere, and the challenge quickly became steep. Accurately identifying a stream of A’s, T’s, C’s, and G’s to spell out a DNA sequence required more finesse than just threading a strand through a pore.
Controlling the speed was particularly tricky, says Akeson. DNA zipped through the pore at more than 100,000 bases per second, blurring the signals from different bases. The team needed an enzyme to grab the DNA and ratchet it through more slowly. Fortunes turned when Akeson’s students tested a new enzyme called Phi29 DNA polymerase. Previous enzymes had secured the DNA strand for just a few milliseconds, but Phi29 held on 40,000 times longer.
The team needed a new pore, too. Hemolysin let DNA through, but its long mushroom stem picked up signals from too many bases at once to distinguish them individually. In 2011, collaborators identified a funnel-shaped bacterial pore called MspA that could pick out all four bases clearly.
Combining the MspA pore with the Phi29 polymerase did the trick. “And there is the first [nanopore] sequence ever seen,” says Deamer reverently, walking over to an image of the resulting trace framed on his office wall. The simplicity of the jagged line, jumping higher or lower as each base passed through, belies the high hurdles overcome by 20 years of tinkering.
From lab to market
The Human Genome Project’s success in February 2001 sparked genomics fever. The cost of sequencing soon dropped dramatically, and the accuracy and speed increased. Scientists could diagnose diseases by comparing patients’ genes with a standard human genome. But sequencing was still expensive and confined to well-funded labs. The nanopore method, if it worked, would offer sequencing in a smaller package—with a much smaller price tag.
In the decade leading up to their first nanopore sequence, Deamer and Akeson weren’t the only ones interested in the approach. A British startup company called Oxford Nanopore also wanted in. They hoped to take the research being done at UCSC, Harvard, and a handful of other labs, and transform it into a marketable handheld sequencer.
In 2007, the chief executives of Oxford Nanopore visited Deamer and Akeson at UCSC and Branton at Harvard, asking to license the patents based on their work. “They brought a mockup of a [handheld] sequencer they thought they’d someday make, and Dave and I both thought that was preposterous,” says Akeson. “We’d pictured sort of a desktop thing.” But they agreed to the partnership.
Commercialization brought a new set of challenges. Oxford Nanopore had received investment funding from U.S. sequencing giant Illumina to develop a different style of nanopore sequencing—but it wasn’t working. When the UCSC team shared the result that enzymes like Phi29 could slow the passage of DNA through the pore, the Oxford Nanopore scientists were so impressed that they abandoned the Illumina-funded line of research.
The consequences were almost disastrous. Illumina managers claimed they should have rights to the new ideas as well. Oxford Nanopore disagreed. The lawsuits that followed triggered a schism between the companies and almost crippled Oxford Nanopore.
Ultimately, the risky maneuver paid off. At a February 2012 conference, the UCSC scientists and their collaborators presented their nanopore sequencing successes. At the same meeting, Oxford Nanopore introduced the MinION—the world’s first instrument that could determine base sequences in single DNA molecules. The announcement unleashed a torrent of excited blog posts and tweets. Scientists called it “mind-boggling” and “a game changer”—if the product could deliver what it promised.
 |
Photo: Laurel Hamers |
A MinION in David Deamer’s lab at UC Santa Cruz. |
The DNA democracy
Oxford Nanopore’s much-hyped announcement didn’t spur instant success. It took two more years to work through the bugs and release the product. But today, hundreds of research teams use MinIONs through an early-access program. The pores and enzymes used in the MinION aren’t the same ones developed at UCSC, but the concepts behind them are similar.
The MinION’s electronics are all contained inside a replaceable piece called a flow cell that sits inside the outer box. A black square less than a centimeter on each side contains 2,048 nanopores; software chooses 512 that seem to be working best. After isolating DNA from cells, researchers use a pipette to place a drop of the solution onto that square. The pores grab single molecules of DNA and ratchet them through, gradually unwinding the double helix. The MinION spits hundreds of raw sequences at once into a computer program, where they can later be analyzed.
Dozens of the devices lie scattered around Akeson and Deamer’s MinION research lab at UCSC, a windowless room that resembles a spacious walk-in closet. A few are festooned with yellow cartoon character stickers—the mischievous Minions from the 2010 film Despicable Me.
Because it’s small and cheap, the MinION is already going places traditional sequencers can’t. “You put the sample on the sequencer, and five minutes later you start seeing answers to the questions you’re asking,” says Miten Jain, one of Akeson’s graduate students. That makes it useful for real-time tracking of environmental conditions or public health, because scientists searching for one set of genes or a disease-related mutation don’t need to read the entire genome.
The advantage became clear during the 2015 Ebola outbreak in West Africa. The blood of infected patients contains clues to which strain of a virus they’ve picked up—information that enables researchers to trace its spread. Field scientists often can’t reconstruct that map until months later, when they get data back from their home lab. But during the outbreak, a team from the University of Birmingham, U.K., used MinIONs to sequence DNA overnight from patients in rural clinics in Guinea. The rapid response helped public health workers allocate resources more wisely and predict the disease’s path.
|
Laurel Hamers interviews Nature journalist and SciCom lecturer Erika Check Hayden, who reported from West Africa at the peak of the Ebola virus outbreak in late 2014. Click on image to play. |
In July 2016, these capabilities reached Earth orbit. A SpaceX rocket launched the MinION to the International Space Station, where scientists hope it will help them monitor microbes that might colonize counters or lurk in the water. The current six-month delay as samples are sent back to Earth for regular sequencing makes the data too old, says Sarah Wallace, a microbiologist at NASA’s Johnson Space Center in Houston. Immediate sequencing also would enable better health care for astronauts on longer missions, such as travel to Mars.
Even in the lab, the MinION has a few advantages over other massive sequencing machines. It can read hundreds of thousands of bases at a time, making piecing together long genomes much simpler. Plus, the MinION is cheap enough for smaller labs to own—and to use as a teaching tool. Geneticist Sophie Zaaijer of Columbia University recently co-taught a course using the MinION. Letting students sequence DNA gives them a better understanding of the science behind their results, she believes. “They really appreciated the fact that they saw the different steps in the process,” she says.
However, the MinION’s accuracy still lags behind that of its huge competitors. For some uses, like identifying single mutations in a gene to assess someone’s risk of developing breast cancer, that accuracy is critical.
Plus, biological materials can be finicky. Cell membranes “are very delicate, they break easily, and that’s still one of the limitations in the MinION,” says Branton. “Not all of the pores are working all the time.” Plus, pores in biological membranes wear out; researchers must replace the flow cell after a few samples. Branton’s group is now trying to develop more resilient nanopores made from graphene. This “solid state” approach hasn’t yet worked, but Branton and Deamer remain optimistic.
Still, for now, the MinION is the only nanopore sequencer available. And the thick layer of patents covering the technology means it’s likely to stay that way for a while, according to Deamer. (While he and his collaborators do receive some money for the patents they developed, the payout is split among many inventors so that “nobody gets rich,” he says.)
Despite his success, Deamer isn’t relaxing. With his original nanopore doodle safely tucked away in a notebook, he has a long list of new ideas taped to his office file cabinet. One of them takes him back to where it all began: He’s now using the MinION to continue his search for how life first emerged from a mess of membranes stewing in primordial volcanic pools.
© 2016 Laurel Hamers / UC Santa Cruz Science Communication Program
Top
Biographies
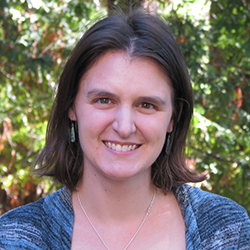
Laurel Hamers
B.A. (biology) Williams College
Internship: Science News
In elementary school, a book on the lonely and impoverished lives of famous poets smashed my dream of someday becoming a writer. I didn’t piece it back together until my senior year of college, when I realized that my appreciation of well-crafted sentences outstripped my aptitude for wrangling fruit flies or crunching data. Plus, the scope of scientific discovery was so great that I couldn’t imagine confining myself to one small corner of it through a research career.
After graduation, my biology degree led me to a job writing press releases—for a physics organization. The words and symbols in the papers I read were in a new language, but I found it satisfying to manipulate them into something resembling English. I’m now hooked on sharing the elegance of science, without using iambic pentameter.
Laurel Hamers’s website
. . . . . . . . . . . . . . . . . . . . . . . . . . . . . . . . . . . . . . . . . . . . . . . . . . .
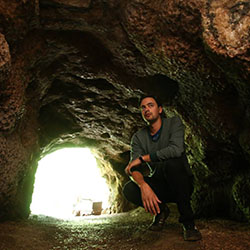
Jay Rasgorshek
B.F.A (narrative art) Minneapolis College of Art and Design
Internship: Raymond M. Alf Museum of Paleontology (Claremont, CA)
My biggest goal has always been to tell compelling stories through my art. I am fascinated with the relationship between the world that surrounds me and the world that I create in my head and on paper. This is why I strived for a BFA in narrative art from MCAD. With this degree and the certification from this program, I am interested in creating metaphors that can easily explain and sell a specific concept to the public that would otherwise be completely lost.
Jay Rasgorshek’s website
Top |