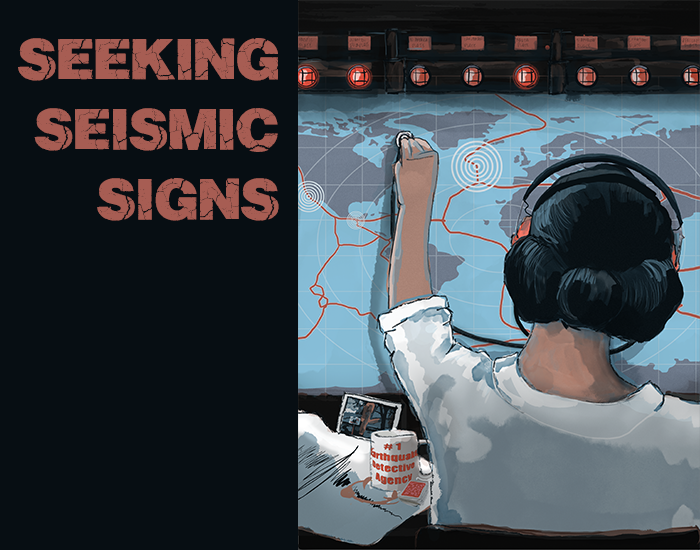
|
Illustration: Kylie Duthie |
Earthquake science has come a long way, but are we any closer to predicting them? Erin E.A. Ross shakes it down. Illustrated by Kylie Duthie and Natalie Renier.
On March 11, 2011, something strange happened in the skies over Japan. Satellites designed to detect bursts of electrical activity from solar flares and other “space weather” recorded a sudden surge of electrons. Forty minutes later, a devastating magnitude 9.0 earthquake off Japan’s eastern coast rocked the country. More than 16,000 people died in the Tohoku earthquake and its resulting tsunami, which swamped the Fukushima nuclear power plant and swept away entire towns.
Geophysicist Kosuke Heki of Hokkaido University in Sapporo, Japan, believes the satellite signal was no coincidence. He has found negative charges hovering in the atmosphere 20 to 40 minutes before 11 major earthquakes around the globe, he says, and he’s searching for more.
Heki and a handful of other researchers say these patterns are precursors: detectable signs that appear before earthquakes. Deciphering these atmospheric irregularities may one day hold the key to predicting temblors—or so their advocates claim.
People started hunting for such precursors soon after earthquake studies began. Anecdotal accounts of pre-earthquake phenomena, such as animals behaving strangely, go back as far as written records. Each generation has identified potential precursors: increases in radon gas, earthquake swarms, magnetic pulses in the ground, “earthquake lights” rising into air. When precursors fail to become predictors, the dedicated researchers keep hope alive. They just need more data and more time, they say, and surely a consistent pattern will emerge.
Many seismologists view the hunt for earthquake predictors as hopeless but harmless. But some publicly question the value of these pursuits. “This has been going on forever,” says seismologist Robert Geller of the University of Tokyo. “It’s like crabgrass. You weed it all out and it comes back again.”
The supposed atmospheric signals are the latest in a long line of failed attempts, Geller says. And in his opinion, trying to predict earthquakes takes attention and funding away from a more grounded goal: preparing communities for the next inevitable disaster.
Shaky history
Earthquake prediction never seemed more likely than it did on a Monday in early September 1975, when millions of people opened their mailbox to an image of Earth torn in two. A lightning-bolt-shaped fault line split the iconic red border of TIME magazine. The headline cried, “Forecast: Earthquake.” In 4,349 words, journalist Frederic Golden argued that so much progress had been made in seismology that scientists would soon not just forecast temblors, but control them.
Golden won the American Institute of Physics Science Writing Award for his story, which featured China’s alleged prediction of the magnitude 7.3 Haicheng earthquake in 1975. According to the Chinese government, the prediction gave them time to evacuate people, saving tens of thousands of lives.
But the next year, an unpredicted magnitude 7.8 earthquake killed more than 240,000 people in Tangshan, China, one of the deadliest natural disasters in history. Many seismologists today agree that a unique combination of factors made the Haicheng prediction possible—and unlikely to be repeated.
In hindsight, it’s easy to see why the forecasting furor of the 1970s arose. The field of seismology was riding the momentum of the recently validated theory of plate tectonics, which posited that Earth’s crust is broken into vast slabs, or plates, that slowly shift. Where two plates grind past each other, tension builds and earthquakes strike. With this new understanding of the forces that shape the planet, mainstream seismologists believed that predicting quakes couldn’t be far off.
“There was a lot of optimism we’d find something that could be used as a predictive tool,” says Golden, speaking over the phone from his home in seismically vulnerable Santa Barbara, California. “Some people even said it had already happened. It was in the air.”
Before the Tangshan disaster, Chinese seismologists claimed to have predicted several earthquakes. Russian scientists were looking into it too, says Golden, and “were talking up a storm.” U.S. researchers had started the Parkfield experiment, where a small earthquake-prone town in central California was wired with a dense array of sensors. On the basis of past patterns, researchers calculated Parkfield would experience a magnitude 6 earthquake every 22 years or so; the next one was due in 1993. Seismologists hoped to find detectable changes in the mighty San Andreas fault before the earthquake. But when it finally arrived, the quake was ten years overdue—and the sophisticated equipment failed to find any clear precursors.
|
Erin Ross drives south to Parkfield, California, where a seismologist and a resident discuss the impacts of the nation’s longest-running earthquake monitoring experiment. Click on image to play. |
For Geller, the Parkfield failure was a nail in the prediction coffin. “The more we do research in seismology, the more we learn how complex earthquakes are, and the more we understand why earthquake prediction is so difficult,” he says.
Researchers with the U.S. Geological Survey, which ran most of the Parkfield experiments, agree that the attitudes of most earth scientists did shift.
“I think the fact that we didn’t see anything at Parkfield is significant,” says USGS geophysicist Andrew Michael, who worked there. Like Geller, Michael doesn’t think we’ll find earthquake precursors any time soon. The USGS made the right decision, he says, when the agency shifted its focus away from earthquake prediction and toward projects to help reduce hazards—like developing new building standards.
Still, Michael feels we shouldn’t stop people from speculating entirely. “I think we’re all trying to do the same thing: save lives,” he says. “But you can also save lives by telling people how and where to build better buildings.”
From the ground up
There’s a simple reason seismologists haven’t found earthquake precursors yet, according to Friedemann Freund of NASA’s Ames Research Center and San Jose State University: They refuse to look outside of seismology.
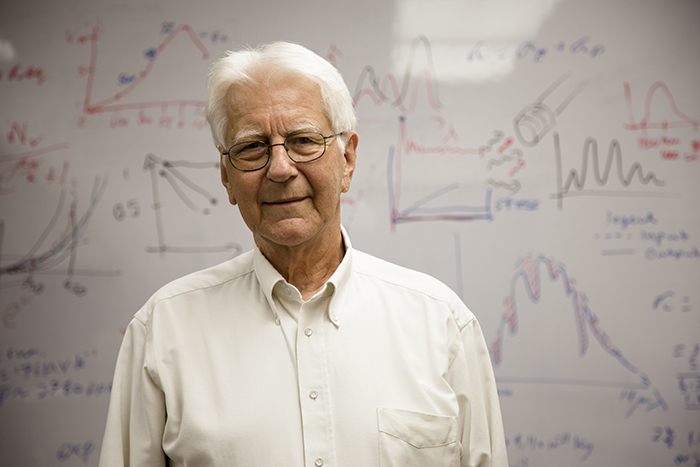 |
Photo: Erin E.A. Ross |
Geophysicist Friedemann Freund. |
|
“My work is against the grain. I am in this strange, wonderful position to be a maverick,” Freund says, leaning back in his chair and grinning expansively, arms held wide. Currently a geophysicist, Freund began his academic career as a chemist and fell into seismology by accident. His big breakthrough, as he calls it, came in 1976 when he was studying his first passion: impurities in crystals.
Freund speaks with his hands and has a fondness for props. He crosses to a cabinet and pulls out a giant framed poster of a blue magnesium oxide crystal. “I grew it myself,” he says proudly. “It has very little impurities.”
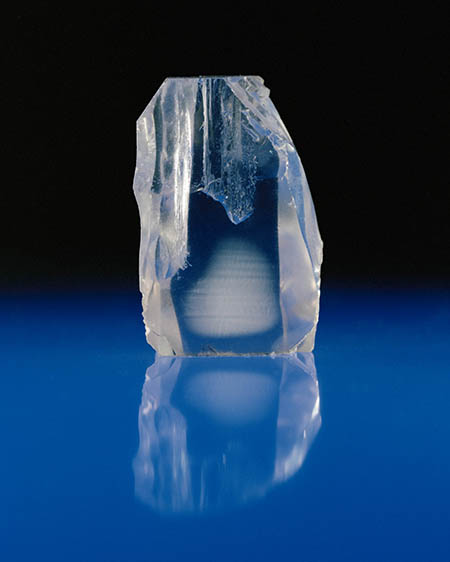 |
Photo courtesy of Friedemann Freund |
Freund’s magnesium oxide crystal. |
|
Crystals that pure don’t occur in nature, so Freund did what any good scientist would do: He put it through all kinds of tests. He heated it to 5,000˚F and chilled it well below freezing. Then he decided to squeeze it. It shattered, but it gave Freund the idea to stress magnesium contained in sturdier rock. When he applied immense pressure to one end of a rock, he detected a massive positive charge at the other end.
“And that’s when I realized that rocks can become sources of electricity,” Freund says. He has since stressed multiple kinds of rock, mostly basalt, and measured those charges again and again. His work led to Freund’s “positive-hole” hypothesis.
Positive charges should show up a short distance from rocks under pressure in nature, Freund believes. The best place to look for stressed rocks, he says, is along an earthquake fault before it breaks.
Freund conjectures that positive holes in such rocks lead to many of the claimed earthquake precursors. The forces can trigger bursts of visible light, he says, which might explain reports of “earthquake lights.” The infrared anomalies sometimes recorded from space before a fault breaks might be from the built-up energy. Freund also cites research showing that some animals react to positively charged air, which might explain reports of dogs, horses, and lab mice acting strangely before earthquakes.
Moreover, Freund believes these positive ground charges are strong enough to rise up through the atmosphere, pulling electrons down from the ionosphere far overhead. Freund has followed the work of Heki and his colleagues, and he’s excited to see his theories applied practically.
Freund has suggested for years that earthquakes cause electric charges, but mainstream seismologists have not made room for him. “They do not understand the physics of positive holes,” he says.
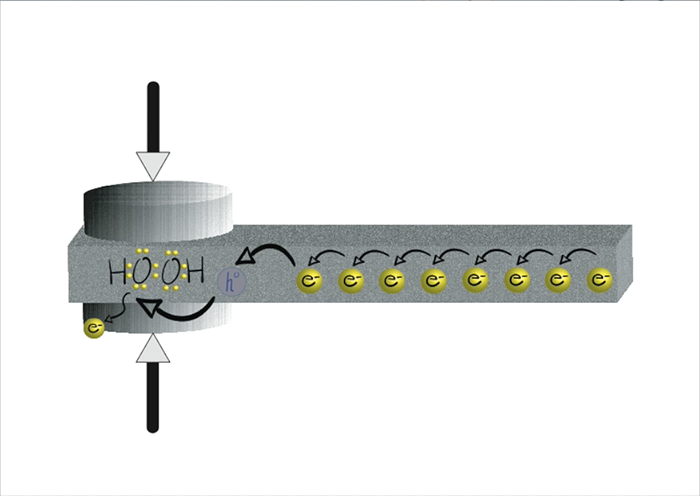 |
Animation: Erin E.A. Ross |
A short animation explains Friedemann Freund’s hypothesis of “positive holes” in stressed rocks. Click on image to play. |
But according to seismologist Thorne Lay at UC Santa Cruz, positive hole physics isn’t the problem. It’s earthquake physics. “Just because there’s stress on a fault doesn’t mean an earthquake is coming,” Lay says. “Sometimes faults relieve stress without shaking the ground.” Seismologists have documented many such “slow-slip” earthquakes, which can unfold gently for years.
Freund believes such earthquakes explain false positives: when a precursor was seen, but no earthquake followed. But Geller and others believe this same phenomenon makes earthquakes fundamentally unpredictable.
Freund relishes the controversy that follows his work. He keeps a document with an 1818 quote by German philosopher Arthur Schopenhauer on his computer desktop. In 64-point font, it reads, “All truth goes through three stages. First, it is ridiculed. Second, it is violently opposed. Third, it is accepted as being self-evident.”
Quest for evidence
Freund and Heki are not alone in searching for pre-earthquake signals in the atmosphere. Geophysicist Sergey Pulinets of the Space Research Institute in Moscow is Freund’s erstwhile colleague and now friendly rival. Like Heki, he has detected electron changes in the ionosphere before earthquakes—but several days prior, not just hours. He believes these patterns are caused by radon gas. Radon is an electrically conductive gas present in small amounts in the ionosphere. Pulinets and others think spiderweb cracks in Earth’s crust release detectable amounts of radon before earthquakes.
Freund dismisses radon’s ability to charge the atmosphere (“There’s just not enough of it!” he proclaims). Whether these atmospheric patterns arise from Freund’s rock stressing, Pulinets’s radon, or an as-yet-undescribed mechanism, the electrical charges far above Earth change constantly. Solar energy bombards the ionosphere, creating a layer of ions with rapidly changing electron counts. Against this ever-fluctuating backdrop, Heki and others search for reliable signs. But sensing seismic signals amidst the loud staccato of ionospheric noise is a steep challenge.
“I still have much research to do before predicting earthquakes with these signals,” says Heki. “It is still premature.”
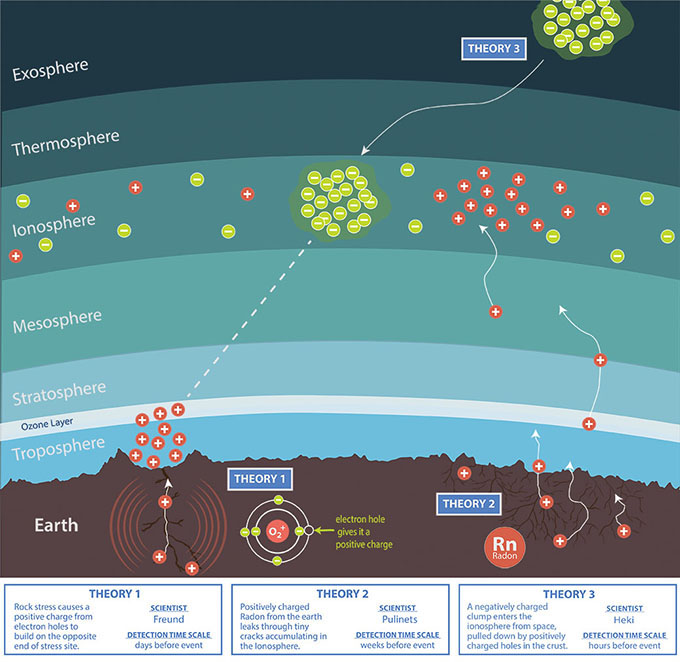 |
Graphic: Natalie Renier |
Scientists have proposed several controversial ideas about electrical patterns that might precede large earthquakes. Click on image to see larger version. |
Heki hopes his research will demystify how these anomalies happen. The first task is determining whether the charge was set off by a force from space or from the ground. How a negative charge moves is the key, Heki explains. If it arrives from space, it travels through the ionosphere and usually moves south from the Arctic. But anomalies from earthquakes are more stationary, he maintains.
Heki is using satellite data to map these ionospheric patterns. He thinks a computer model eventually will pinpoint the origins of each one. “But it is very difficult to do so in real time,” he says.
A matter of selection
But finding signals “in real time” is essential for saving lives. Instead, today’s scientists work after the shaking stops, rushing to find some kind of identifying signal that might have acted as a warning. Critics caution that conducting science in this retrospective way leaves too much wiggle room.
“Frankly, I’d be surprised if these guys weren’t finding associations,” says Philip Stark, a statistician at UC Berkeley who studies earthquake forecasts and predictions. It’s a basic statistical problem, he explains. Between chemical changes in groundwater, electromagnetic pulses on the ground, and electric signals in the atmosphere, you’re bound to find something strange before an earthquake if you look hard enough.
Even when researchers are only investigating one potential pre-earthquake signal, like electron changes in the sky, the data set is vast, Stark says. Dozens of satellites monitor space weather at any given time, so researchers have a lot of places to find atmospheric precursors.
“It’s selection bias,” says Geller of the University of Tokyo. “If you’re cherry-picking a large enough data set, you can find anything.”
There is also massive variation in how the purported anomalies appear: days in advance, less than an hour before the quake strikes, right over the epicenter, or appearing to drift on the wind dozens or hundreds of miles away.
Critics have jumped on the retrospective studies and their undefined results. Geophysicist Fabrizio Masci of the National Institute of Geophysics and Vulcanology in L’Aquila, Italy, has reviewed the methodology of a handful of these studies. He failed to find evidence that earthquakes sparked the ionospheric anomalies. Other researchers have scoured records as well, with similarly unfruitful results. One laboratory tried, and failed, to reproduce Freund’s rock-stressing results—although Freund says this was due to differences in study design.
To Stark and Geller, ionospheric precursors are no different from any other supposed earthquake signal. No matter how many scientists argue that we cannot predict these deadly events, Stark believes people will keep looking. “Human beings are hard-wired to find patterns,” he says. “That’s very much part of our evolutionary biology.”
The adherents of earthquake precursors are well aware of the criticism they face. They know they’ll have to overcome staunch opposition for their theories to be accepted. Freund does not intend to stop his pursuit. As he puts it, “It’s just too important.”
_______________________________________________________________
Sidebar: Cloudy with a Chance of Earthquakes

Mainstream seismologists shun earthquake predictions, but agencies like the U.S. Geological Survey issue quake “forecasts” in the form of hazard maps and assessments. The maps chart the faults in a region, assigning a percentage likelihood that a given-size earthquake will rupture each fault within, say, 30 years.
Many factors go into producing these maps, explains geophysicist Andrew Michael, who works in hazard assessment at the USGS. They examine a region’s past history of earthquakes, as well as the shape and structure of the fault line.
“If you look at a fault’s earthquake history, you can forecast the largest earthquakes forward in time with a statistical model known as the Poisson model. It assumes that earthquakes are independent events that happen randomly,” explains Michael.
However, a prominent statistician questions the theory behind such assessments. “The numbers are essentially useless,” says Philip Stark of UC Berkeley. “It’s bad statistics.”
Earthquakes aren’t random or regular, counters Stark. Rather, they clump together in time. “You can think of hazard assessments like a casino game,” he says. “If you draw a blank card, there’s no earthquake. If you draw an 8, there’s a magnitude 8. Seismologists debate how many 8s there are in the deck and whether cards are replaced after they’re drawn. But earthquakes aren’t like a casino game at all.”
As Stark and others have noted, the strength of a statistical model relies on its predictive ability—and that’s where hazard assessments fall flat. Just because a fault has behaved one way in the past, they say, doesn’t mean it will behave the same way in the future. Critics of the maps cite the 2011 Tohoku earthquake and tsunami in Japan that killed more than 16,000 people. Few seismologists believed that fault zone could produce an earthquake above magnitude 8. But it reached magnitude 9—more than 30 times as powerful.
“Stark and others say hazard assessment professionals need to change their models. I would argue that we’re already doing that,” says Michael. The most recent assessment for California takes earthquake clustering into account. The models are also becoming increasingly sophisticated. Some try to gauge the speed a fault is moving or measure deformation in the land around it. Other models allow faults to link together, producing larger earthquakes.
Still, Stark cautions, we should find a better way to communicate earthquake risk to the public and governments—one that steps away from statistical forecasts. “People want numbers. People love numbers,” says Stark. “But as soon as you give them a number, they stop thinking.”
To Michael, it all comes down to a debate between different schools of statistical thought. “It’s like a religious divide over what probabilities mean. But the engineers who develop our building codes understand these issues and still want these numbers. We can deal with the philosophical differences later.”
© 2016 Erin E.A. Ross / UC Santa Cruz Science Communication Program
Top
Biographies
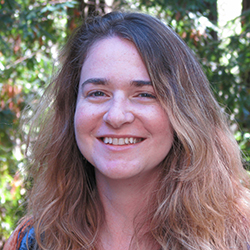
Erin E.A. Ross
B.A. (biology) Clark University
Internships: National Institute of General Medical Sciences, National Public Radio
When I was in kindergarten, I loved to tell my mom what I would be when I grew up. On Mondays, I would be a wildlife photographer. Tuesdays, an ecologist. Wednesdays, a geologist. As good mothers do, mine explained that most people don’t change careers daily. As good children do, I informed her that she was totally wrong. She just needed to wait and see.
College didn’t make my career path clearer. I loved everything I did, from writing to studying mosquito ecology to exhibiting my photography. After graduation, I started doing science communication at the New England Aquarium and the Boston Museum of Science. As I discovered the joy of weaving stories for my audiences, I learned something else: When you share science for your career, you get to be something new every day.
Erin E.A. Ross’s website
. . . . . . . . . . . . . . . . . . . . . . . . . . . . . . . . . . . . . . . . . . . . . . . . . . .
Kylie Duthie
B.F.A. (studio art) University of California, Davis
Internship: Del Monte Forest Conservancy, Pebble Beach, CA
Kylie Duthie is an illustrator living on the Monterey Peninsula. She received an associate degree in studio art and transferred to UC Davis to study printmaking, receiving her BFA in studio art. She will intern for the Del Monte Forest Conservancy to help raise awareness of the endangered endemic botanical species of the Del Monte forest.
Kylie Duthie’s website
. . . . . . . . . . . . . . . . . . . . . . . . . . . . . . . . . . . . . . . . . . . . . . . . . . .
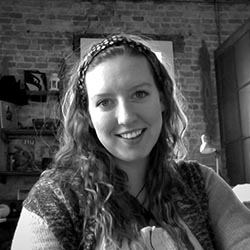
Natalie Renier
B.Sc. (aquatic science) University of Wisconsin-
La Crosse
Internships: Moss Landing Marine Laboratory and
The Ridges Sanctuary
Natalie is a science illustrator with a special interest in aquatic ecosystems. After obtaining a degree in biology she decided to pursue her dream of attending the Science Illustration program at California State University Monterey Bay. During internships and beyond she hopes to continue creating art in the service of science.
Natalie Renier’s website
Top |