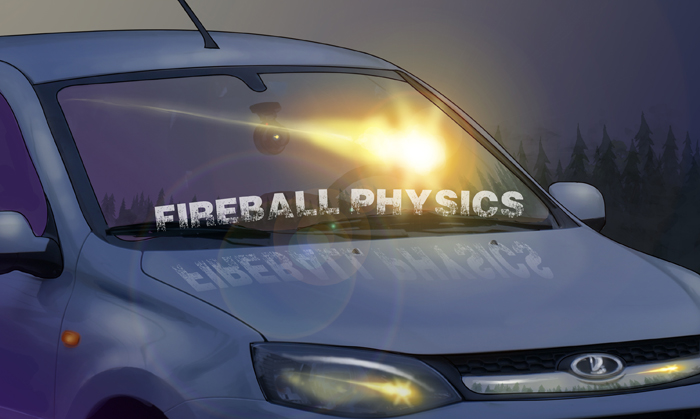
|
Illustration: Mary Williams |
The best-documented meteor was a blast for scientists to reconstruct. Nicholas St. Fleur retraces the Russian shock waves. Illustrated by Mary Williams.
Peter Jenniskens paused as he reached for the front door to his hotel in Chelyabinsk, Russia. Only a few jagged shards of thick glass stuck out from the wooden frame. He collected the fragments—the first of many clues that the NASA scientist would gather to understand the gigantic fireball that erupted over the city three weeks earlier.
“I had in my hands pieces of glass that were destroyed by an asteroid impact. How unbelievable is that?” Jenniskens says.
The shards were tiny pieces of a puzzle assembled by Jenniskens and an international team about the most startling cosmic encounter in decades. The Chelyabinsk meteor on February 15, 2013, came without warning. It streaked across the dawn sky in a dazzling display of red and orange trailed by two columns of puffy smoke. Then with a flash of light, it exploded mid-air. Moments later, a fierce shockwave rocked the one million people below.
No one died, but the blast injured 1,600 people—some through flying glass like the shards Jenniskens collected. Evidence about the intense physics of the blast came from patterns of damaged buildings, sound recordings in the atmosphere, and pieces of the rock itself—including a 1,400-pound chunk fished from a nearby lake. But the best clues came from security cameras and car-mounted dash cams that captured footage of the meteor as it shot through the atmosphere and exploded—making it, by far, the best-documented space impact in history.
“We know that these events can be very violent, but to have this happen in our lifetime in this densely populated of an area was incredible,” Jenniskens says.
But to illuminate the extraordinary physics of a real fireball, the team had to spend weeks on the ground in Siberia, tracing hints of the blast’s imprints at every turn.
Examining the tapes
A tall, skinny man whose face brightens at the word “meteorite,” Jenniskens had led an expedition to recover a space rock that crash-landed in Sudan in 2008. He also tracked down meteorites from the 2012 Sutter’s Mill impact in the Sierra Nevada. Like nearly everyone else, he was alerted to the Chelyabinsk fireball through headlines on the news and a flood of footage uploaded to YouTube. Thanks to social media, what would have been an isolated explosion became the airblast heard, and seen, around the world.
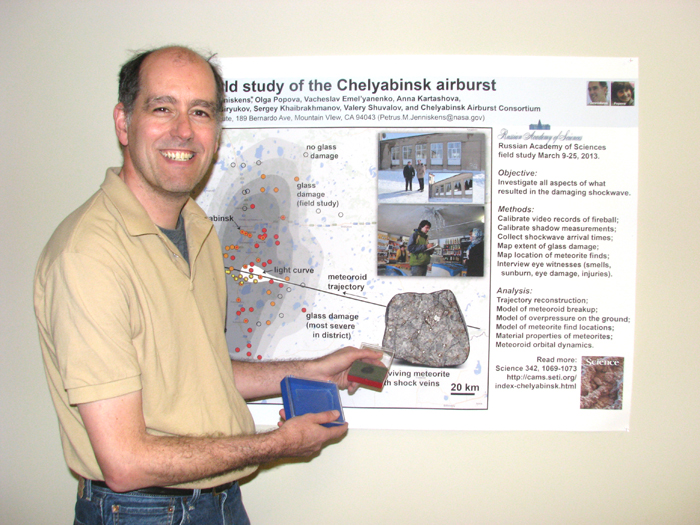 |
Meteor expert Peter Jenniskens of NASA holds two keepsakes from the February 2013 fireball over Chelyabinsk, Russia: a shard of blown-out glass and a fragment of the meteorite itself. (Photo: Nicholas St. Fleur) |
By sheer coincidence, scientists were preparing for a much larger asteroid called 2012 DA14 to hurtle past Earth that same day. But the Chelyabinsk fireball was a surprise. The asteroid was relatively small, and its arrival was masked by the sun’s glare. It evaded detection of every satellite and radar when it pierced the atmosphere.
Low frequency, long-distance vibrations called infrasound that emanated from the shockwave clued scientists in to what had happened. Infrasound stations across the world detected the waves. The closest detectors in Kazakhstan reported an explosion equivalent to the energy released by 500 kilotons of TNT, bigger than most small nuclear blasts. In 1908 an impact perhaps 10 to 100 times more powerful had flattened a huge tract of trees near Tunguska, Siberia. But that astral invasion was poorly documented. Chelyabinsk was the first blast captured and shared in the social networking era. With a few clicks, footage was seen by millions. It provided a wealth of evidence for remote scientists like Jenniskens to delve into immediately.
For the next few weeks, he pored over the films. “Here's a perfect video,” Jenniskens says, playing a clip from the dash cam of a car stopped at a traffic sign. “When the driver arrives—for just a few frames—you see the fireball appear. And from that moment on you get a beautiful record of the whole trajectory.”
Jenniskens and his team combed through more than 400 such videos. Each one provided a different perspective and viewing angle of the event. The videos created the foundation for his investigation. When Jenniskens found one that met his criteria—a still, clear camera shot with points of reference, such as trees or buildings—he downloaded it and extracted its individual frames. He narrowed his search to ten videos that represented the locations he felt he needed to visit.
|
Click on this interactive graphic to see videos used by meteor hunter Peter Jenniskens and Nature authors J. Borovička et al. to reconstruct the Chelyabinsk fireball. Yellow markers indicate where Jenniskens traveled to map the night sky. Blue markers show the locations used by the Nature authors to calculate the trajectory. Blue dots show places visited by both teams. The red line marks the fireball's trajectory, with dots marking key moments. Data from Popova, O. P. et al. in Science 342, 1069–1073 (2013) and Borovička, J. et al. in Nature 503, 235–237 (2013). (Infographic by Nicholas St. Fleur.) |
“The moment I saw the videos, my emotion was that I want to go there,” Jenniskens says. “Ideally I would have liked to have been there when it happened.” He found a host: meteor modeler Olga Popova of the Russian Academy of Sciences’ Institute for Dynamics of Geospheres, who invited Jenniskens to join her team in Chelyabinsk to reconstruct the meteorite’s trajectory.
Many videos caught the meteor’s entrance and exit, but missed its middle moments when the explosion’s intense light blinded the cameras. To determine the rock’s speed, Jenniskens analyzed traffic camera footage that caught moving shadows from the backside of houses as the meteor blew up. This speed, and the asteroid’s path as it punched through the atmosphere, let scientists trace the rock back to its origins in our solar system’s asteroid belt.
Jenniskens knew from his expedition in Sudan that sleuthing this meteor impact on the ground would be no easy task. He needed to visit each site and take a photo of the scenery against the night sky, precisely matching each reference point to triangulate the object’s flight course. The stars, he says, act like a compass for determining the direction of the fireball and deducing its orbit from space. Even small changes when lining up his camera could have big consequences for accuracy.
But unlike his previous ventures, this one was a race against time. The data were rapidly disappearing: glass was being repaired, chunks of meteorites were being recovered, and people’s memories were fading. Three weeks after the fiery impact, Jenniskens embarked for Russia.
Cold case
After landing in bone-chilling Chelyabinsk, where the temperature dropped to 0°F, Jenniskens and his team first investigated a damaged zinc factory. The building’s walls had collapsed following the Chelyabinsk explosion. It was one of more than 7,000 buildings to have its windows blown out by the shockwave.
The team marked the sites where they saw shattered glass to map the physical extent of the damage caused by the shock. “You see the pressure wave not just push against the glass, but also push the whole window frame,” Jenniskens says. “You see people get blown off their feet from the shockwave. You wonder why more people didn’t get hurt.” In a city of a million people, no one died—despite the blast’s intense power. But many injuries did occur when people ran to their windows to see the bright flash of light, just before the blast wave roared across the city.
The team traveled to more than 50 villages on the outskirts of the blast, some more than 50 miles away. They visited local markets at each stop to speak with shop owners, who had spent three weeks discussing the events with customers. The shopkeepers would summarize how the fireball impacted their town. When the team heard other reports of damage, they traveled to those sites and assessed the impact.
“Every person we spoke to had something to say about it. Either they had seen the fireball or they felt the pressure wave coming towards them,” says Jenniskens. “It was really an exceptional event.” The testimonies supplied information beyond what the videos could capture, such as the smells, sounds, and injuries in the blast’s aftermath.
In some cases, Jenniskens met people who were outside during the fireball. Many of them reported smelling sulfur, like after a fireworks display. One coal miner was working in a snowfield right under the blast; he suffered severe sunburns and peeling skin. Jenniskens calls the airburst, which produced brutally bright light, the most exceptional aspect of the entire event.
The team also collected fragments of the meteorites, which they sent back to labs around the world to analyze. Geologist Qing Zhu Yin of UC Davis received a penny-sized fragment. He used intense X-rays to examine the meteorite’s consistency. Its weakly bound layers of minerals and severe fragmenting revealed why it exploded into thousands of pieces of debris. His team also looked at the rock’s magnetic field and determined that it was made of “ordinary chondrite,” a rocky asteroid containing little iron.
“This is one of the bystander witnesses to the formation of the solar system four and a half billion years ago,” Yin says. Most asteroids that hit Earth will be like this one, he notes. Much more rare are solid iron bodies, like the one that gouged Meteor Crater in Arizona 40,000 years ago.
Bursts and blasts
A week before Jenniskens left for Chelyabinsk, physicist Mark Boslough of Sandia National Laboratory in Albuquerque, New Mexico, was already on his way. Boslough, a world authority on air blasts, uses computer models to simulate how fireballs cascade toward the ground—and their potential to kill people. Asteroids that pierce the atmosphere release much of their kinetic energy as pressure, which creates the air blast. The Chelyabinsk meteor provided a natural lab to test Boslough's ideas about how things blow up overhead.
Boslough was one of the first American scientists to find out about the meteor event. He too got the message through social media. An hour after the fireball exploded, a Canadian friend who plays late-night chess online with Russian players shared the news from a site documenting the explosion. Boslough immediately identified it as an airburst.
“It was an adrenaline rush from that moment I saw the first video,” he says. “The first thing I wanted to do was set up a model and start simulating it.”
A few days later, a team from the NOVA television program contacted Boslough to accompany them to Chelyabinsk for three days as their meteorite expert. They wanted to detail the event in a short documentary called “Meteor Strike.” But Boslough had more than just TV on his agenda. Equipped with a camera, tripod, and warm winter clothes, he set out to track down data and learn more about the blast. During the day he filmed with the NOVA crew, but at night Boslough sleuthed with a taxi driver across the countryside, looking for clues.
“I don’t think of it so much as Sherlock Holmes, because he's all about solving mysteries with clues,” Boslough says. “There was never a mystery about what this was. We just wanted to get the best possible data, and we wanted to get it quickly so we could explain all the details surrounding the blast.” In particular, the airburst’s path overhead—created by the asteroid’s trajectory—would help test Boslough’s simulations of explosions above the ground at different angles.
He also used his model to help deduce why the airwave created twin trails of smoke. “When we first saw the airwave split we didn’t know why, but as soon as we started modeling it, it all made sense,” Boslough says. The meteor ignited a horizontal cylinder of hot air. As that buoyant air rose, it split into two horizontal vortices resembling sideways tornadoes.
“Modeling ties everything to physics,” Boslough says. “The way the airwave split into two, the intensity of the shockwave, and the pattern of damage on the ground—all of these are completely explainable with physics, but only if you make the observations.”
Podcast produced by Nicholas St. Fleur. Click on arrow to play.
The unlikely impact
Thousands of miles away in Canada, Boslough’s partner Peter Brown, a meteor scientist from the University of Western Ontario, started analyzing the data Boslough transmitted. Together, they calibrated the blast to characterize the meteorite’s size, energy, and the physics of its impact. It was the first time scientists had such calibrations for an airburst.
“In the first few hours, it was clear to me from looking at the data that this object was way beyond the scale and scope of anything we’d ever seen,” says Brown. Within 12 hours, Brown had made a preliminary estimate that the blast exploded with 420 kilotons of energy. Over the next six months, he used data from infrasound, dash-cam videos, light detectors, and seismic sensors to put that number at 500 kilotons. It had been more than a century since humans had recorded anything that powerful from a piece of space debris.
“I certainly never expected to see an object of this size hit anywhere on the earth, let alone near a city, during my career," Brown says. "It’s just very unlikely.”
Brown, Boslough, Jenniskens, and their colleagues used the deluge of data to pinpoint where the rock came from, decode what it was made of, and trace its destructive path through the sky. Nine months following their trips, the two teams reported a suite of superlatives in the journals Science and Nature: a 12,000-ton, 65-foot-wide space rock had hurtled across the sky at 42,500 miles per hour, bursting 14 miles above the city in a flash of light 30 times brighter than the sun and 30 times more powerful than the Hiroshima atomic bomb.
Brown says the event gives scientists a better sense of the hazard that any given small object poses to our planet. The mild 18-degree angle at which the asteroid entered and then exploded was lucky for Chelyabinsk. Had the rock come at a steeper angle, such 45 degrees, the downward-driving airburst would have had graver impacts on the residents.
It also helps reveal the frequency of such events. Previously touted as a once-in-a-thousand-years event, Chelyabinsk-size impacts are getting their frequencies reevaluated. According to David Morrison, an astronomer at the SETI Institute in Mountain View, California, similar events may strike every 40 to 100 years, on average.
“It makes you question at what size of an incoming object would you want to evacuate or get out of the way and at what size would you say ‘Hey that’s cool, let’s go watch it.’ This was right on the border between those two,” he says.
The blast investigation, says Morrison, helps lay the groundwork for preparing for the next event. Scientists now can use models to run an ensemble of scenarios that vary in meteorite size, impact angle, speed, and other variables, then create risk assessments accordingly.
“It's not that scientists didn’t know this could happen," Morrison says. "Rather, now there’s a broader community concerned about these impacts and saying ‘Hey, we need to understand this.’”
His colleague, Peter Jenniskens, holds two keepsakes from his time in Chelyabinsk: a small, smooth chunk of the meteorite and a thick shard of glass shattered by the shockwave. The pocket-sized souvenirs are pieces to a physics puzzle that he never thought he would be so fortunate to solve.
© 2014 Nicholas St. Fleur / UC Santa Cruz Science Communication Program
Top
Biographies
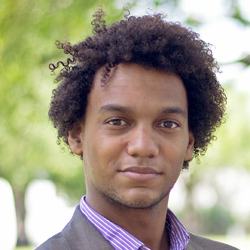
Nicholas St. Fleur
B.S. (biological sciences; minor: communications) Cornell University
Internships: National Public Radio, Scientific American
A magnitude-7.0 earthquake triggered my interest in science writing. The sliding tectonic plates and severe aftershocks were fascinating enough, but the human side of the seismological story inspired me.
I was a first-year premed student when the quake devastated Port-au-Prince, the capital of Haiti—and the city where my parents were born. For the next week I was transfixed as medical correspondents painted a morbid picture of the disease outbreaks and death following the disaster. Though it was unsettling to watch, I found myself captivated by this juxtaposition of medicine and media.
I soon enrolled in a science and health reporting course and developed a passion for storytelling. Only rare science stories have tragedy at their epicenters, but they all have humanity beneath the surface, and I intend to unearth it.
Nicholas St. Fleur's website
. . . . . . . . . . . . . . . . . . . . . . . . . . . . . . . . . . . . . . . . . . . . . . . . . . .
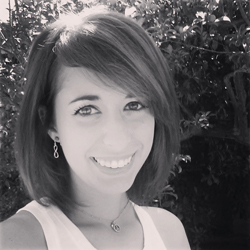
Mary Williams
B.F.A (illustration) Savannah College of Art and Design
Internship: Field Museum of Natural History, Chicago
I had no idea what I wanted to do as a career growing up. My plans fluctuated almost weekly from teaching, to becoming a veterinarian, to studying marine biology or oceanography. Drawing had always been something fun for me to do, but it wasn’t until high school that I realized I could make it into a gratifying, life-long practice. Even then I had no idea I could combine science and nature with illustration. Thankfully all the pieces fell into place, and I’m doing exactly what I love with a wide-open road ahead of me.
Mary Williams's website
Top |